R. Yaari1, K.C. Holdridge1, J. Choi2, M.C. Donohue2, K. Kantarci3, C.R. Jack Jr3, S.M. Zuk3, J.R. Sims1, K.A. Johnson4, P.S. Aisen2, R.A. Sperling4,5, for the A4 Study Team6
1. Eli Lilly and Company, Indianapolis, IN, USA; 2. University of Southern California, San Diego, CA, USA; 3. Mayo Clinic and Foundation, Rochester, MN, USA;
4. Massachusetts General Hospital, Boston, MA, USA; 5. Brigham and Women’s Hospital, Boston, MA, USA; 6. https://a4study.org/a4-study-team/
Corresponding Author: Roy Yaari, Eli Lilly and Company, Lilly Corporate Center, Indianapolis, IN 46285, USA, yaari_roy@lilly.com, +1 317-416-0872
J Prev Alz Dis 2022;4(9):617-624
Published online June 7, 2022, http://dx.doi.org/10.14283/jpad.2022.56
Abstract
BACKGROUND: Screening data from the Anti-Amyloid Treatment in Asymptomatic Alzheimer’s Disease (A4) and Longitudinal Evaluation of Amyloid Risk and Neurodegeneration (LEARN) studies provide a unique opportunity to compare magnetic resonance imaging (MRI) findings such as amyloid-related imaging abnormalities (ARIA) in cognitively unimpaired elderly with and without elevated cerebral amyloid.
OBJECTIVES: To compare screening MRI findings, such as ARIA, in the cognitively unimpaired potential participants of a clinical trial with and without elevated cerebral amyloid.
DESIGN: Cross-sectional analysis of structural MRI findings in screening data from the A4 and LEARN studies.
SETTING: The A4 Study is a multi-center international clinical trial. The LEARN Study is a multi center observational study in the United States.
PARTICIPANTS: Clinically normal older adults (65-85 years) with elevated cerebral amyloid (Aβ+; n = 1250, A4) and without elevated cerebral amyloid (Aβ-; n = 538, LEARN).
MEASUREMENTS: Participants underwent florbetapir positron emission tomography for Aβ+/- classification. A centrally read 3T MRI to assess for study eligibility was conducted on study qualified MRI scanners.
RESULTS: No ARIA-effusions (ARIA-E) was detected on screening MRI in the Aβ+ or Aβ- cohorts. At least one ARIA-H (microhemorrhages [MCH] or superficial siderosis [SS]) was present in 18% of the Aβ+ cohort compared with 8% in Aβ- (P < 0.001). In the Aβ+ cohort, approximately 2% of screening MRIs demonstrated MCH ≥4 compared with 0% in Aβ-. The presence of two apolipoprotein E ε4 (APOEε4) alleles (vs no ε4 alleles) in the Aβ+ cohort increased the odds for presence of MCH (odds ratio [OR] = 2.03; 95% CI, 1.23 to 3.27, P = 0.004). Cortical infarctions (4% vs 0%) and subcortical infarctions (10% vs 1%) were observed at statistically significantly higher prevalence in the Aβ+ cohort compared with Aβ- (P < 0.001). Females showed reduced odds of MCH in the Aβ+ cohort by a factor of 0.63 (95% CI, 0.47 to 0.84, P = 0.002).
CONCLUSIONS: ARIA-E is rare in cognitively unimpaired Aβ+ and Aβ- populations prior to anti-amyloid drug intervention. ARIA-H in Aβ+ was greater than in Aβ- populations.
Key words: Amyloid-related imaging abnormalities, preclinical Alzheimer’s disease, magnetic resonance imaging, florbetapir positron emission tomography.
Introduction
Two types of imaging abnormalities associated with anti-amyloid immunotherapy were first observed in early phase Alzheimer’s disease (AD) dementia trials (1). One type appeared to be consistent with cerebral vasogenic edema, and the other appeared to be consistent with cerebral hemosiderin deposition. These magnetic resonance imaging (MRI) abnormalities have since been associated with multiple amyloid-modifying immunotherapies. In 2011, an academic and industry leader workgroup termed these findings amyloid-related imaging abnormalities (ARIA), with presumed shared underlying pathophysiological mechanisms related to amyloid clearance from brain vasculature or parenchyma, and categorized the two types as follows: “vasogenic edema or effusion” (ARIA-E), as seen on fluid-attenuated inversion recovery (FLAIR) MRI, and hemosiderin deposition (ARIA-H) (2). ARIA-H is subdivided into the following two categories: microhemorrhages (MCH), which are small, <10 mm signal hypointensities on T2* weighted/ gradient refocused echo (GRE) MRI scans, and superficial siderosis (SS), which occur in the subarachnoid space lining the cortical surface, ≥10 mm (3).
Although associated with amyloid-modifying therapy, ARIA is also seen in the natural history of AD, but at lower rates. In patients with AD dementia without exposure to anti amyloid therapy, ARIA-E prevalence estimates range from <0.1% to 0.8% (4-7) and ARIA-H prevalence estimates range from 9.2% to 33% (6-11). In a community-dwelling population of 60- to 79-year-old individuals, the ARIA-H prevalence was 19.8% (12) and 17% in age ≥50 (mean age 69.8), with a positive amyloid positron emission tomography (PET) standardized uptake value ratio (SUVR) (13). In population cohorts of cognitively unimpaired individuals, a positive correlation was found between SUVR on amyloid PET scan and number of MCH (10, 14). Apolipoprotein E ε4 (APOEε4) is a risk factor for both ARIA-E and ARIA-H in the sporadic AD population (2).
The Anti-Amyloid Treatment in Asymptomatic AD (A4) Study is a secondary prevention trial testing solanezumab in preclinical AD (15), and the Longitudinal Evaluation of Amyloid Risk and Neurodegeneration (LEARN) Study is a companion study to A4 that enrolled individuals who met inclusion criteria for A4 on cognitive and medical measures but did not have evidence of elevated cerebral amyloid (Figure 1). Screening data from the A4 and LEARN studies allow for comparison of MRI findings including ARIA in a large cognitively unimpaired cohort both with and without elevated cerebral amyloid.
Methods
The A4 Study (NCT02008357) is being conducted at 67 clinical trial sites in the United States, Canada, Japan, and Australia in cognitively unimpaired individuals with elevated Aβ as determined by florbetapir PET. Participants first underwent an initial clinic screening visit to assess cognitive and medical eligibility. Eligible participants then underwent florbetapir PET imaging at a second screening visit. If the PET demonstrated elevated cerebral amyloid, then a brain MRI for eligibility was conducted prior to study drug randomization in the A4 Study. If the PET did not demonstrate elevated cerebral amyloid, then participants were referred to the LEARN Study (NCT02488720), in which an MRI was also conducted for eligibility. This analysis included all screening MRIs, regardless of whether the participants were eligible to enter the study.
Participants who completed screening for the A4 and LEARN studies were aged 65 to 85 years and considered cognitively unimpaired based on a global Clinical Dementia Rating score of 0, Mini-Mental State Exam score of 25 to 30, and Logical Memory II subscale delayed paragraph recall of the Wechsler Memory Scale-Revised score of 6 to 18. Key exclusion criteria for participants were use of AD medications, unstable anxiety or depression, or other unstable medical conditions, although participants with treated hypertension, diabetes, and other common medical ailments were permitted. The 4486 participants who met these criteria then underwent florbetapir amyloid PET imaging.
A4 Screening Amyloid PET Imaging
Florbetapir F 18 PET was acquired approximately 50 minutes after injection of 10 mCi of florbetapir F 18. Amyloid eligibility (elevated cerebral amyloid and eligible to continue in A4 screening vs not elevated and ineligible for A4 but eligible for LEARN) was assessed using an algorithm combining both quantitative SUVR methodology and qualitative visual read performed at a central laboratory. Mean SUVR using a whole cerebellar reference region of ≥1.15 was used to define elevated amyloid (Aβ+). An SUVR between 1.10 (estimated centiloid value of 24.1) and 1.15 (estimated centiloid value of 33.3) was considered to be Aβ+ only if the visual read was considered positive by consensus from two independent readers. Individuals who did not meet these amyloid PET criteria were considered not elevated or amyloid negative (Aβ-) and were eligible to screen for the LEARN Study until the target size of 500 LEARN participants was reached.
A4 and LEARN MRI
A 3T MRI to assess for study eligibility was conducted on study qualified MRI scanners and were centrally read to help assess eligibility and describe screening MRI findings including presence of ARIA-E, ARIA-H, and evidence of other cerebral pathology. Included sequences were three-dimensional T1 MPRAGE, axial T2*/GRE, axial T2 FLAIR, axial diffusion weighted MRI, and axial T2 FSE/TSE. The A4 and LEARN studies did not have the same central readers and had slightly different variables for recording MRI findings (Appendix A). The central readers were aware of amyloid eligibility status at the time the MRIs were read.
Statistical Analyses
Screening MRI findings from the Aβ+ and Aβ- cohorts were summarized and compared with Fisher’s exact test (binary findings) or Mann–Whitney U test (continuous or ordinal findings). The association between MRI findings and continuous potential risk factors were assessed with boxplots and Spearman’s rank correlation. The association between MRI findings and categorical variables were assessed with histograms and proportional odds models. Stepwise (forward and backward) model selection by Akaike information criterion (AIC; (16)) was used to build regression models of MRI findings with covariates for demographics and potential risk factors separately within the Aβ+ and Aβ- cohorts. Predictors considered in the model selection were as follows: age, gender, Preclinical Alzheimer’s Cognitive Composite (PACC) score, individual PACC components (Free and Cued Selective Reminding (FCSRT), Digit Symbol Substitution Test (DSST), Logical Memory IIa (LMIIa), Mini-Mental State Examination (MMSE)), Cognitive Function Index (CFI), Alzheimer’s Disease Cooperative Study-Activities of Daily Living (ADCS-ADL), number of APOE4 alleles (0,1,2), presence of APOE4 alleles (0, >0), florbetapir PET SUVr, concurrent medication of antiplatelet/antithrombotic agent (ATC2C-B01), medical history of hypertension, medical history of diabetes and medical history of both hypertension and diabetes. Note that PACC and individual components were not retained in the same final model. Logistic regression was used for binary findings and the proportional odds model for ordinal findings. Logistic regression models were checked for partial separation and infinite maximum likelihood estimates (17, 18). All analyses were conducted using R version 3.6.2.
Results
Baseline demographics were well balanced across the Aβ+ or Aβ- cohorts (Table 1). No ARIA-E was detected on screening MRI in the Aβ+ or Aβ- cohorts (Table 1). At least one ARIA-H (MCH or SS) was present in 18% of the Aβ+ cohort compared with 8% in Aβ- (P < 0.001). In the Aβ+ cohort, approximately 2% of screening MRIs demonstrated MCH ≥4 (exclusionary for A4 Study participation) compared with 0% in Aβ-. MCH, cortical infarctions, and subcortical infarctions were observed at statistically significantly higher prevalence in the Aβ+ cohort compared with Aβ-. In the Aβ+ cohort, 11 of 18 participants with SS also had at least one MCH, whereas in the Aβ- cohort, there were no reports of SS combined with MCH. No significant correlations were observed between continuous risk factors (PACC, Total Recall, Delayed Total Recall, MMSE, Digit Symbol, CFI, ADCS-ADL, FBP PET SUVr and age in years) and number of MCH in either Aβ+ or Aβ- cohorts. The presence of two APOEε4 alleles in the Aβ+ cohort increased the odds for presence of MCH (odds ratio [OR] = 2.0267 [95% confidence interval (CI) 1.2336 to 3.2669, P = 0.004]) compared with no APOEε4 alleles (Table 2). The presence of one APOEε4 allele did not increase the odds of MCH in the Aβ+ cohort (OR = 0.9834 [95% CI, 0.7198 to 1.3464, P = 0.916]). The Aβ- cohort had two APOEε4 homozygotes—too few to analyze, but aggregating into carrier status did not increase the odds of MCH (OR = 0.726 [95% CI, 0.3064 to 1.5294, P = 0.429]). Females also showed reduced odds of MCH in the Aβ+ cohort by a factor of 0.6286 (95% CI, 0.4701 to 0.8402, P = 0.002) with equal percentages of APOEε4 carriers in both the female (425/733 = 58%) and male (312/517 = 59%) subgroups. In the Aβ+ cohort, subcortical infarction was associated with an increased odds of MCH by a factor of 2.1799 (95% CI, 1.4159 to 3.2935, P < 0.001); the Aβ- cohort did not have a sufficient number of cortical (N = 2) or subcortical infarctions (N = 3) to assess (Table 1).
All data expressed as N (%) unless otherwise noted. P-values for screening MRI findings are from Fisher’s exact test. Participants with MRI scan date but with NA entries were considered to have «0» or «No» entries. Abbreviations: A4 = Anti-Amyloid Treatment in Asymptomatic Alzheimer’s Disease; APOEε4 = apolipoprotein E ε4; ARIA = amyloid related imaging abnormalities; ARIA-E = ARIA-effusions; LEARN = Longitudinal Evaluation of Amyloid Risk and Neurodegeneration; MRI = magnetic resonance imaging; N = number of participants; NA = not applicable; SD = standard deviation.
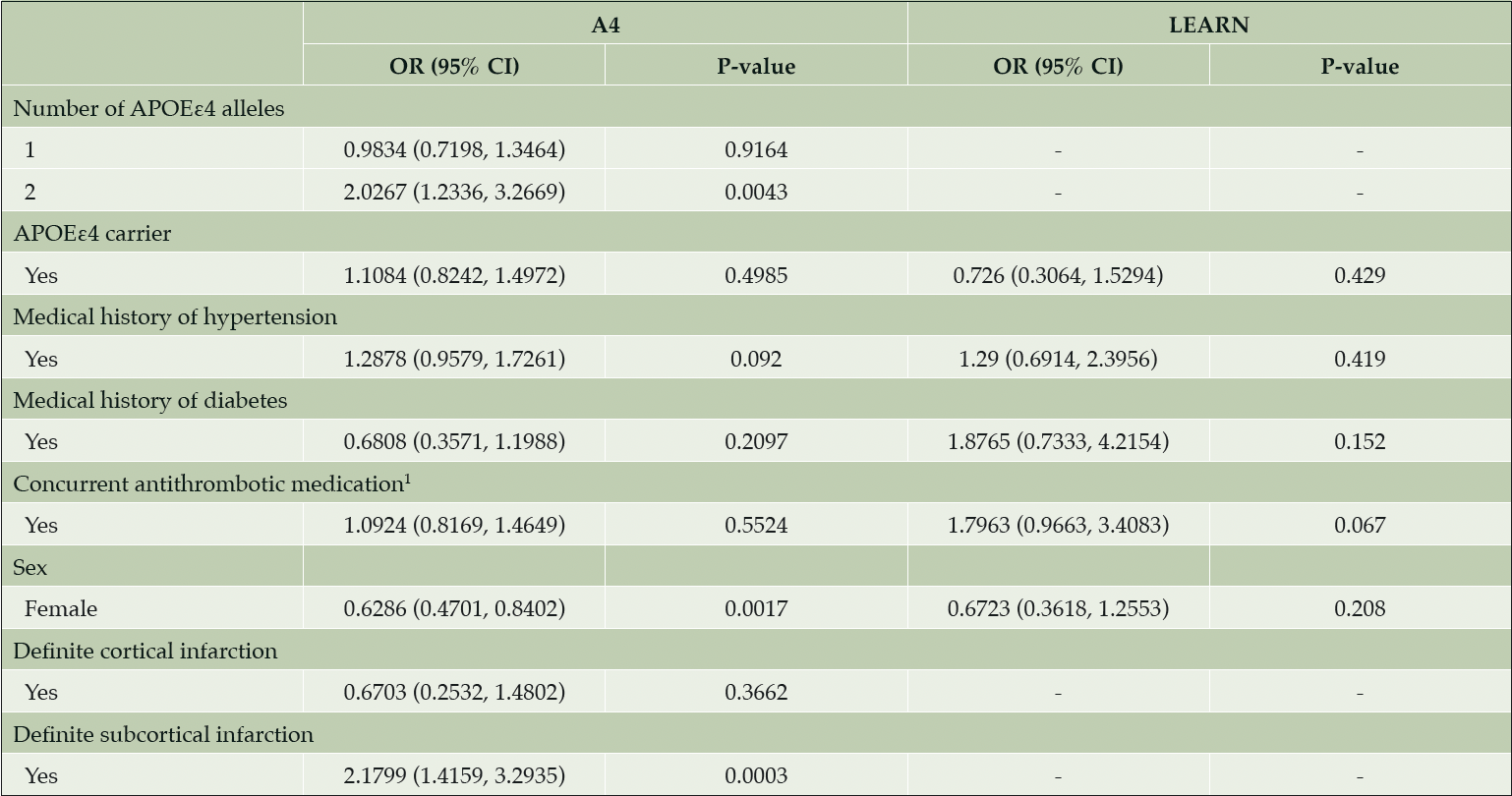
Table 2. Parameter estimates from proportional odds regression models of MCH and categorical risk factors
1. Antithrombotic agents defined as ATC-B01 = Anatomical Therapeutic Chemical Classification System Code, such as vitamin K antagonists, platelet aggregation inhibitors, and Direct factor Xa inhibitors. Abbreviations: A4 = Anti-Amyloid Treatment in Asymptomatic Alzheimer’s Disease; APOEε4 = apolipoprotein E ε4; CI = confidence interval; LEARN = Longitudinal Evaluation of Amyloid Risk and Neurodegeneration; MCH = microhemorrhage; OR = odds ratio.
A stepwise model selection (forward and backward) in the Aβ+ cohort identified that worse CFI score, greater age, and having two APOEε4 alleles increased the risk for number of MCH. Better PACC score and female sex decreased the risk for number of MCH (Table 3). Having one APOEε4 allele and history of diabetes were also in the model, but the effects were not significant. In the Aβ- cohort, no variables were identified as statistically significant predictors of MCH (Table 3). Results from stepwise model selection with MCH as a binary variable for both the Aβ+ and the Aβ- cohorts yielded similar results. The logistic regression models showed no evidence of separation or unreliable estimates due to divergence of the maximum likelihood estimates.
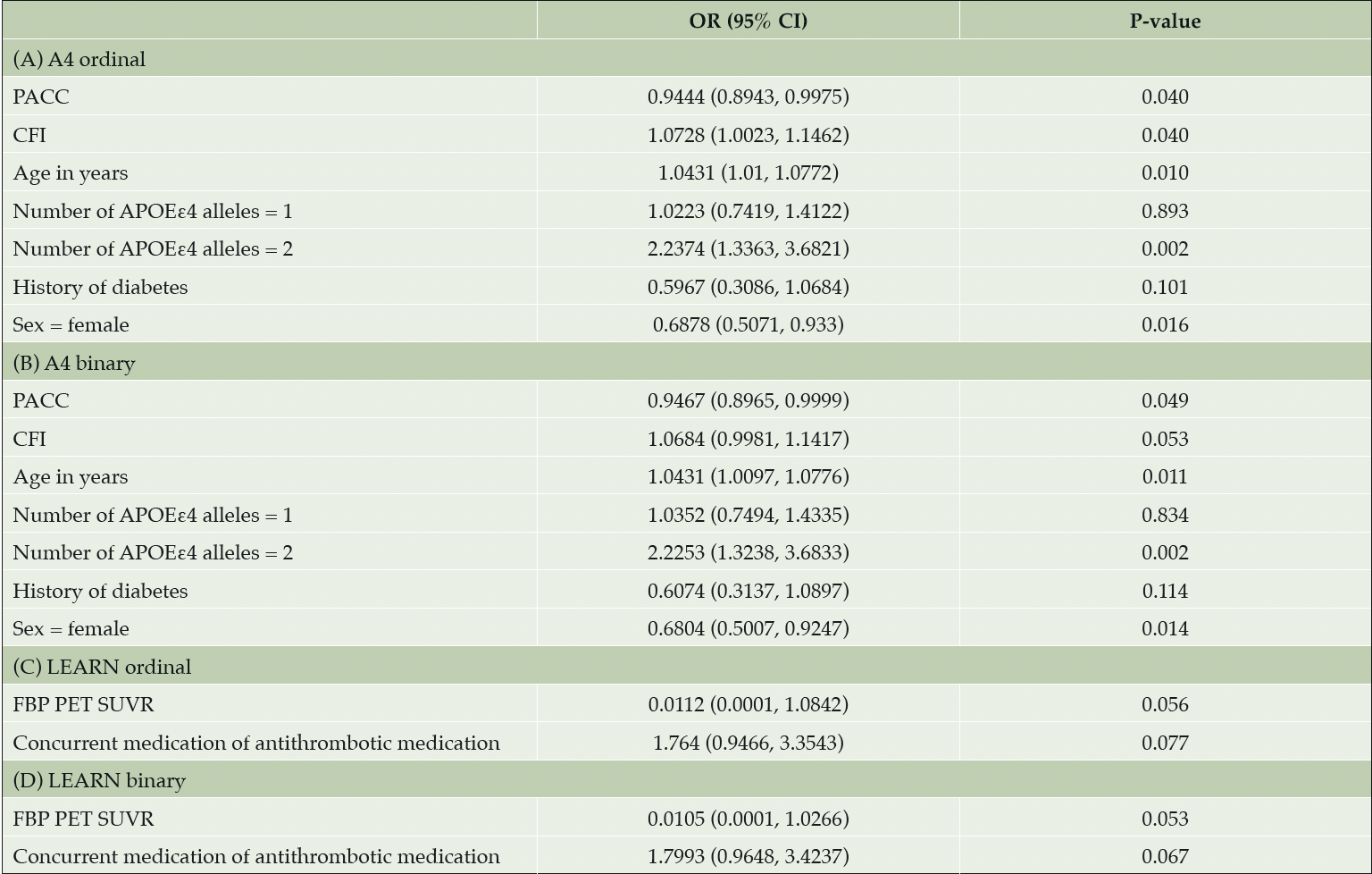
Table 3. Predictors for number of MCH ordinal and binary in a stepwise model selection (forward and backward) in Aβ+ and Aβ- cohorts
Notes: Variables included in the model were age, gender, PACC, CFI, ADCS-ADL, number of APOEε4 alleles (0, 1, 2), presence of APOEε4 alleles (0, >0), florbetapir PET SUVR, medical history of hypertension, concurrent medication of antiplatelet/antithrombotic agent, and medical history of diabetes. Abbreviations: A4 = Anti-Amyloid Treatment in Asymptomatic Alzheimer’s Disease; Aβ+ = elevated amyloid; Aβ- = amyloid negative; ADCS-ADL = Alzheimer’s Disease Cooperative Study—Activities of Daily Living; APOEε4 = apolipoprotein E ε4; CFI = cognitive function index; CI = confidence interval; FBP = florbetapir; LEARN = Longitudinal Evaluation of Amyloid Risk and Neurodegeneration; OR = odds ratio; PACC = Preclinical Alzheimer’s Cognitive Composite; PET = positron emission tomography; SUVR = standard uptake value ratio.
Discussion
The absence of ARIA-E on screening Aβ+ MRI comports with the known low prevalence of spontaneous ARIA-E in the symptomatic AD population.
The ARIA-H prevalence in the Aβ+ cohort of 18% comports with historical data in populations with AD dementia and community-dwelling elderly populations in which amyloid status is unknown. The ARIA-H prevalence of 8% in the Aβ- cohort is lower than that in community-dwelling populations in which the amyloid status is not known, likely due to the estimate that approximately 30% of the elderly population has elevated amyloid (15, 19-21). However, cerebrovascular disease is associated with MCH, and participants with a history of substantial cerebrovascular disease were excluded from the study, which may have contributed to the lower prevalence. Additionally, despite extensive efforts to recruit a diverse population to participate in both the A4 and LEARN studies, the majority of participants were white (94% in A4 and 93% in LEARN) and non-Hispanic or Latino ethnicity (96% in both A4 and LEARN) which may have contributed to, and further reduced, the generalizability of the findings. Although the numbers were relatively small, the greater prevalence of multiple MCH (MCH ≥2) in the Aβ+ cohort compared with the Aβ- cohort also suggests a correlation of greater likelihood of multiple MCH with elevated cerebral amyloid (Aβ+ cohort [5.4%] vs Aβ- cohort [2.6%], P = 0.009). These values are comparable with multiple MCHs reported in a population-based study of non-dementia individuals ages 60 to 69 of 3%, but less than reported in older age groups: 10% aged 70 to 79, and 19% aged 80 and above (9).
The presence of a single APOEε4 allele, generally considered to be an MCH risk factor, did not appear to have a significant correlation with MCH, suggesting that a single APOEε4 allele may not be an MCH risk factor in the preclinical AD population. However, having two APOEε4 alleles approximately doubled the odds of presence of MCH in the Aβ+ cohort, and AIC selected APOEε4, but not amyloid PET SUVR. This finding is corroborated in a cohort of a community “nondemented” population that found a single APOEε4 allele to have no significant association with development of new MCHs, whereas two APOEε4 alleles had a significant association (22). In the Aβ+/- combined cohort, AIC selected both APOE and amyloid PET status, suggesting their independent predictive value. The Aβ- cohort had few APOEε4 homozygotes. Additionally, the reduced OR in females (OR = 0.6286) in the Aβ+ cohort suggests the possibility of sex-based risk of developing MCH in people who are cognitively unimpaired and Aβ+, as a greater incidence of MCH in men has been reported in a population based study of elderly adults without dementia (9). Of note, the OR for female sex in the Aβ- cohort was similar (OR = 0.6723) though not statistically significant. Use of an antiplatelet agent or anticoagulant did not significantly increase the odds of MCH in either cohort; however, a prior meta-analysis from 37 relevant studies with a sample size of 20,988 reported that MCH were more frequent in people using an antiplatelet agent (pooled OR = 1.21; 95% CI, 1.07 to 1.36; P = 0.002) (23).
In the stepwise model for predictors of MCH in the Aβ+ cohort, a better score on the PACC, a sensitive cognitive composite test, reduced odds of MCH (OR = 0.9444, P < 0.040) and an increase in CFI (indicating worsening function) increased odds of MCH (OR = 1.0728, P < 0.040), suggesting a correlation between worsened scores on clinical scales and MCH in Aβ+.
Additionally, in Aβ+, an increase in age, having two APOEε4 alleles, and history of hypertension increased odds of MCH, which has been previously reported in population studies (12). The statistically significantly reduced odds of MCH in the Aβ+ females has not previously been reported and could indicate sex differences in pathophysiology of MCH when amyloid is present.
The 1% prevalence of SS in the Aβ+ cohort is consistent with the natural history as previously reported, with an estimated prevalence of 1.43% in a community population >69 years (24). The same study reported an association between SS and increased cerebral brain amyloid SUVR, supporting CAA as a possible etiology. The difference in SS prevalence between the Aβ+ and Aβ- cohorts further supports this hypothesis. In the Aβ+ cohort, more than half of participants with SS also had MCH (11 of 18 participants); concomitant MCH were not observed in the Aβ- cohort with SS (0 of 2 participants). These results are consistent with findings in population studies: in the Mayo Clinic Study of Aging, 3 of 13 individuals with SS had concomitant MCH (24), and 7 of 7 individuals with SS had concomitant MCH in the Rotterdam Scan Study (25). These results also suggest that Aβ+ individuals have a greater likelihood for concomitant MCH and SS.
The notable elevation in screening infarction (both cortical and subcortical) in the Aβ+ cohort relative to the Aβ- cohort suggests amyloid as a risk factor for stroke, and the significantly increased odds of MCH with subcortical stroke in Aβ+ supports a common etiology, possibly due to cerebral amyloid angiopathy, which also has a strong association with APOEε4 (26). An increased risk of ischemic stroke with MCH in a community-dwelling population with unknown amyloid status has previously been reported (hazard ratio = 1.93; 95% CI, 1.25 to 2.99) (27, 28).
The prevalence of incidental cerebral infarction (combined cortical and subcortical) on MRI of 12% in the community-dwelling Lothian Birth Cohort 1936 with a mean age of 72.5 years (29) is similar to the prevalence in the Aβ+ cohort, but the prevalence in the Aβ- cohort is much lower, suggesting a correlation between cerebral infarction and elevated amyloid.
Some features of the design of the A4 and LEARN studies should be taken into consideration, which could limit conclusions from the findings. The protocol included a population of generally healthy older individuals, excluding participants with substantial vascular disease including people with significant prior infarcts or cerebral hemorrhage. In post-screening MRIs, the MRI reviewers may observe findings that were present at screening, but that could not be confidently classified due to motion or other artifacts, and therefore were not recorded as a definite finding at the time of the screening MRI. For example, the central reader may have reported no MCHs on a screening MRI, but on a subsequent MRI, the reviewer may identify an MCH that in retrospect was present at screening, and then amend the original MRI report to include the finding of an MCH; conversely, a later review could remove a previously reported finding. The A4 Study has at least four scheduled post-screening MRIs in the double-blind period, and in the LEARN Study, one scheduled post-screening MRI, which could lead to ascertainment bias.
A relatively small subset of the over 3000 Aβ- individuals from the A4 screening process underwent MRI, as the LEARN Study was only open to enroll approximately 540 individuals and finished enrollment prior to the A4 Study. Additionally, in both the A4 and LEARN studies, 3T MRIs were used, which are more sensitive to MCHs and other findings than 1.5T, which then complicates comparability to prior studies using 1.5T. Another limitation is that screening MRIs for the A4 and LEARN studies (thus, the Aβ+ and Aβ- cohorts) were reviewed by different central MRI readers with slightly different categories for reporting incidental infarctions. Although in LEARN, cortical infarcts were quantified, “subcortical infarcts” was not a LEARN variable, and for the purposes of this analysis, the free text field for “other” was reviewed to determine whether subcortical infarcts were present, which may have resulted in an underreporting of subcortical infarcts in the Aβ- cohort.
Although the screening findings of ARIA-H and incidental infarcts were higher in the Aβ+ cohort, very few of these findings met exclusion criteria for participation in the studies. Given the exploratory nature of this study, future research may further identify differences on MRI between cognitively unimpaired Aβ+ versus Aβ- populations, and associated risk factors. While also verifying the findings herein, these future studies may also help determine if risk factors for baseline ARIA-H will be risk factors for treatment emergent ARIA with anti-amyloid monoclonal antibodies, potentially providing context for safety monitoring in preclinical AD populations.
Funding: The A4 Study is a secondary prevention trial in preclinical Alzheimer’s disease, aiming to slow cognitive decline associated with brain amyloid accumulation in clinically normal older individuals. The A4 Study is funded by a public private philanthropic partnership, including funding from the National Institutes of Health-National Institute on Aging (U19AG010483; R01AG063689), Eli Lilly and Company, Alzheimer’s Association, Accelerating Medicines Partnership, GHR Foundation, an anonymous foundation, and additional private donors, with in-kind support from Avid, Cogstate, Albert Einstein College of Medicine, US Against Alzheimer’s Disease, and Foundation for Neurologic Diseases. The companion observational Longitudinal Evaluation of Amyloid Risk and Neurodegeneration (LEARN) Study is funded by the Alzheimer’s Association and GHR Foundation. The A4 and LEARN studies are led by Dr. Reisa Sperling at Brigham and Women’s Hospital, Harvard Medical School, and Dr. Paul Aisen at the Alzheimer’s Therapeutic Research Institute (ATRI), University of Southern California. The A4 and LEARN studies are coordinated by ATRI at the University of Southern California, and the data are made available through the Laboratory for Neuro Imaging at the University of Southern California. The participants screening for the A4 Study provided permission to share their de-identified data in order to advance the quest to find a successful treatment for Alzheimer’s disease.
Acknowledgements: We would like to acknowledge the dedication of all the participants, the site personnel, and all of the partnership team members who continue to make the A4 and LEARN studies possible. The complete A4 Study Team list is available on a4study.org/a4-study-team. The authors would like to acknowledge Deirdre Hoban, PhD, an employee of Eli Lilly and Company, for project management support, and strategic and scientific communication expertise.
Disclosures: RY, KCH and JRS are full-time employees and minor shareholders of Eli Lilly and Company. RY has received funds from Eli Lilly and Company for attending meetings and or/travel. JC has received research funding from NIH and Janssen. MCD has received research funding from NIH and Janssen; consulting fees from Roche; has participated on a Data Safety Monitoring Board or Advisory Board for the University of California, San Diego and has a spouse who is a full-time employee of Janssen. KK has received research funding from NIH and ADDF; consulting fees from Biogen; support for attending meetings and/or travel from Alzheimer’s Association; has participated on a Data Safety Monitoring Board or Advisory Board for Takeda; has a leadership or fiduciary role in other board, society, committee or advocacy group for Alzheimer’s Association (unpaid) and NIH and has received research materials from Avid Radiopharmaceuticals. CRJ Jr. has received research funding from NIH, the GHR Foundation, and the Alexander Family Alzheimer’s Disease Research Professorship of the Mayo Clinic; participated on a Data Safety Monitoring Board or Advisory Board for Roche (unpaid); has served as a speaker for Eisai, and consulted for Biogen, but he receives no personal compensation from any commercial entity. SMZ has no conflicts of interest to declare. KAJ has received research funding from NIH; consulting fees from Novartis and has participated on a Data Safety Monitoring Board or Advisory Board for Cerveau (unpaid). PSA has received research funding from NIH, Alzheimer’s Association and Eli Lilly and Company for the A4 Trial; research agreements with Janssen, Eli Lilly and Company and Eisai; research funding from NIA, the Alzheimer’s Association and FNIH and consulting fees from Biogen, Roche, Merck, Abbvie, Immunobrain Checkpoint, Rainbow Medical and Shionogi. RAS has received research funding from NIH, Alzheimer’s Association and Eli Lilly and Company for the current study; research funding from Eli Lilly and Company, Janssen, NIA, Alzheimer’s Association; consulting fees from AC Immune, Acumen, Alnylam, Cytox, Janssen, JOMDD, Nervgen, Neuraly, Neurocentria, Oligomerix, Prothema, Renew, Shionogi, Vigil Neuroscience, Ionis, Biogen, Eisai and Roche; honoraria for Harvard HUBWeek Panel (2018), Japan JSNM (2018), Marc Diamond UT Southwestern Symposium (2019), University of Chicago DOM Weekly Grand Rounds Seminar Series (2020), Virtual Rhode Island IDeA Symposium (2020) and Virtual IMPACT-AD Course (2020) and support for attending meetings and/or travel from Duke Lectureship (2018), Alzheimer Research Roundtable (2018), ADPD Symposium (2019), University of Tokyo Roundtable (2019), Biogen: ARIA State of the Art meeting (2019), University of Pittsburgh Distinguished Scientist Lecture (2019), University of Cincinnati Lurie Lecture (2019), UC Irvine Distinguished Lecture on the Brain (2020), Virtual Rhode Island IDeA Symposium (2020), Adler Foundation Symposium San Diego (2020), UC Irvine Lecture (2020)
Ethical standards: All studies were conducted in accordance with the Declaration of Helsinki, the International Conference on Harmonization Good Clinical Practice guideline, and local regulatory requirements. The study protocols were approved by an independent ethics committee or institutional review board at each study site. All patients provided written consent before the start of the study. All studies were registered at ClinicalTrials.gov: A4 Study (NCT02008357) and LEARN Study (NCT02488720).
Open Access: This article is distributed under the terms of the Creative Commons Attribution 4.0 International License (http://creativecommons.org/licenses/by/4.0/), which permits use, duplication, adaptation, distribution and reproduction in any medium or format, as long as you give appropriate credit to the original author(s) and the source, provide a link to the Creative Commons license and indicate if changes were made.
References
1. Salloway S, Sperling R, Gilman S, Fox NC, Blennow K, Raskind M, et al. A phase 2 multiple ascending dose trial of bapineuzumab in mild to moderate Alzheimer disease. Neurology. 2009;73(24):2061-70.
2. Sperling RA, Jack CR, Jr., Black SE, Frosch MP, Greenberg SM, Hyman BT, et al. Amyloid-related imaging abnormalities in amyloid-modifying therapeutic trials: recommendations from the Alzheimer’s Association Research Roundtable Workgroup. Alzheimers Dement. 2011;7(4):367-85.
3. Brashear HR, Ketter N, Bogert J, Di J, Salloway SP, Sperling R. Clinical Evaluation of Amyloid-Related Imaging Abnormalities in Bapineuzumab Phase III Studies. J Alzheimers Dis. 2018;66(4):1409-24.
4. Carlson C, Estergard W, Oh J, Suhy J, Jack CR, Jr., Siemers E, et al. Prevalence of asymptomatic vasogenic edema in pretreatment Alzheimer’s disease study cohorts from phase 3 trials of semagacestat and solanezumab. Alzheimers Dement. 2011;7(4):396-401.
5. Raman MR, Wiste HJ, Senjem ML, Ward CP, Jack CR, Jr., Kantarci K. Spontaneous amyloid-related imaging abnormalities in a cognitively normal adult. Neurology. 2014;83(19):1771-2.
6. Sperling R, Salloway S, Brooks DJ, Tampieri D, Barakos J, Fox NC, et al. Amyloid-related imaging abnormalities in patients with Alzheimer’s disease treated with bapineuzumab: a retrospective analysis. Lancet Neurol. 2012;11(3):241-9.
7. Carlson C, Siemers E, Hake A, Case M, Hayduk R, Suhy J, et al. Amyloid-related imaging abnormalities from trials of solanezumab for Alzheimer’s disease. Alzheimers Dement (Amst). 2016;2:75-85.
8. Goos JD, Henneman WJ, Sluimer JD, Vrenken H, Sluimer IC, Barkhof F, et al. Incidence of cerebral microbleeds: a longitudinal study in a memory clinic population. Neurology. 2010;74(24):1954-60.
9. Graff-Radford J, Botha H, Rabinstein AA, Gunter JL, Przybelski SA, Lesnick T, et al. Cerebral microbleeds: Prevalence and relationship to amyloid burden. Neurology. 2019;92(3):e253-e62.
10. Kantarci K, Gunter JL, Tosakulwong N, Weigand SD, Senjem MS, Petersen RC, et al. Focal hemosiderin deposits and beta-amyloid load in the ADNI cohort. Alzheimers Dement. 2013;9(5 Suppl):S116-23.
11. Ketter N, Brashear HR, Bogert J, Di J, Miaux Y, Gass A, et al. Central Review of Amyloid-Related Imaging Abnormalities in Two Phase III Clinical Trials of Bapineuzumab in Mild-To-Moderate Alzheimer’s Disease Patients. J Alzheimers Dis. 2017;57(2):557-73.
12. Poels MM, Vernooij MW, Ikram MA, Hofman A, Krestin GP, van der Lugt A, et al. Prevalence and risk factors of cerebral microbleeds: an update of the Rotterdam scan study. Stroke. 2010;41(10 Suppl):S103-6.
13. Graff-Radford J, Lesnick T, Rabinstein AA, Gunter J, Aakre J, Przybelski SA, et al. Cerebral microbleed incidence, relationship to amyloid burden: The Mayo Clinic Study of Aging. Neurology. 2020;94(2):e190-e9.
14. Yates PA, Sirisriro R, Villemagne VL, Farquharson S, Masters CL, Rowe CC, et al. Cerebral microhemorrhage and brain beta-amyloid in aging and Alzheimer disease. Neurology. 2011;77(1):48-54.
15. Sperling RA, Donohue MC, Raman R, Sun CK, Yaari R, Holdridge K, et al. Association of Factors With Elevated Amyloid Burden in Clinically Normal Older Individuals. JAMA Neurol. 2020;77(6):735-45.
16. Sakamoto Y, Ishigoruro M, Kitgawa G. Akaike Information Criterion Statistics. Reidel D, editor. Dordrecht, The Netherlands: Springer Nature Customer Service Center LLC; 1986. 316 p.
17. Kosmidis I, Schumacher D. detectseparation: Detect and Check for Separation and Infinite Maximum Likelihood Estimates. R package version 0.1. . 2020.
18. Lesaffre E, Albert A. Partial separation in logistic discrimination. . Journal of the Royal Statistical Society: Series B (Methodological). 1989;51(1):109-16.
19. Dubois B, Epelbaum S, Nyasse F, Bakardjian H, Gagliardi G, Uspenskaya O, et al. Cognitive and neuroimaging features and brain beta-amyloidosis in individuals at risk of Alzheimer’s disease (INSIGHT-preAD): a longitudinal observational study. Lancet Neurol. 2018;17(4):335-46.
20. Jack CR, Jr., Wiste HJ, Weigand SD, Therneau TM, Knopman DS, Lowe V, et al. Age-specific and sex-specific prevalence of cerebral beta-amyloidosis, tauopathy, and neurodegeneration in cognitively unimpaired individuals aged 50-95 years: a cross-sectional study. Lancet Neurol. 2017;16(6):435-44.
21. Jansen WJ, Ossenkoppele R, Knol DL, Tijms BM, Scheltens P, Verhey FR, et al. Prevalence of cerebral amyloid pathology in persons without dementia: a meta-analysis. JAMA. 2015;313(19):1924-38.
22. Poels MM, Ikram MA, van der Lugt A, Hofman A, Krestin GP, Breteler MM, et al. Incidence of cerebral microbleeds in the general population: the Rotterdam Scan Study. Stroke. 2011;42(3):656-61.
23. Qiu J, Ye H, Wang J, Yan J, Wang J, Wang Y. Antiplatelet Therapy, Cerebral Microbleeds, and Intracerebral Hemorrhage: A Meta-Analysis. Stroke. 2018;49(7):1751-4.
24. Pichler M, Vemuri P, Rabinstein AA, Aakre J, Flemming KD, Brown RD, Jr., et al. Prevalence and Natural History of Superficial Siderosis: A Population-Based Study. Stroke. 2017;48(12):3210-4.
25. Vernooij MW, Ikram MA, Hofman A, Krestin GP, Breteler MM, van der Lugt A. Superficial siderosis in the general population. Neurology. 2009;73(3):202-5.
26. Rannikmae K, Samarasekera N, Martinez-Gonzalez NA, Al-Shahi Salman R, Sudlow CL. Genetics of cerebral amyloid angiopathy: systematic review and meta-analysis. J Neurol Neurosurg Psychiatry. 2013;84(8):901-8.
27. Akoudad S, Portegies ML, Koudstaal PJ, Hofman A, van der Lugt A, Ikram MA, et al. Cerebral Microbleeds Are Associated With an Increased Risk of Stroke: The Rotterdam Study. Circulation. 2015;132(6):509-16.
28. Bokura H, Saika R, Yamaguchi T, Nagai A, Oguro H, Kobayashi S, et al. Microbleeds are associated with subsequent hemorrhagic and ischemic stroke in healthy elderly individuals. Stroke. 2011;42(7):1867-71.
29. Sandeman EM, Hernandez Mdel C, Morris Z, Bastin ME, Murray C, Gow AJ, et al. Incidental findings on brain MR imaging in older community-dwelling subjects are common but serious medical consequences are rare: a cohort study. PLoS One. 2013;8(8):e71467.