J. Barakos1,2, D. Purcell1,2, J. Suhy1, S. Chalkias3, P. Burkett3, C. Marsica Grassi4, C. Castrillo-Viguera4, I. Rubino4, E. Vijverberg5
1. Bioclinica Inc., Newark, CA, USA; 2. California Pacific Medical Center, San Francisco, CA, USA; 3. Moderna Inc., Cambridge, MA, USA; 4. Biogen Inc., Cambridge, MA, USA; 5. Alzheimer Center Amsterdam, Department of Neurology, Amsterdam Neuroscience, Vrije Universiteit Amsterdam, Amsterdam UMC, Netherlands.
Corresponding Author: Jerome Barakos, Medical Imaging, Neuroscience, Bioclinica, 7707 Gateway Boulevard, 3rd Floor, Newark, CA 94560, USA. Tel: 415 515-3058, Fax: 415 753-9363. E-mail: barakosmd@gmail.com
J Prev Alz Dis 2022;
Published online February 2, 2022, http://dx.doi.org/10.14283/jpad.2022.21
Abstract
Amyloid-related imaging abnormalities (ARIA) are adverse events reported in Alzheimer’s disease trials of anti-amyloid beta (Aβ) therapies. This review summarizes the existing literature on ARIA, including bapineuzumab, gantenerumab, donanemab, lecanemab, and aducanumab studies, with regard to potential risk factors, detection, and management. The pathophysiology of ARIA is unclear, but it may be related to binding of antibodies to accumulated Aβ in both the cerebral parenchyma and vasculature, resulting in loss of vessel wall integrity and increased leakage into surrounding tissues. Radiographically, ARIA-E is identified as vasogenic edema in the brain parenchyma or sulcal effusions in the leptomeninges/sulci, while ARIA-H is hemosiderin deposits presenting as microhemorrhages or superficial siderosis. ARIA tends to be transient and asymptomatic in most cases, typically occurring early in the course of treatment, with the risk decreasing later in treatment. Limited data are available on continued dosing following radiographic findings of ARIA; hence, in the event of ARIA, treatment should be continued with caution and regular monitoring. Clinical trials have implemented management approaches such as temporary suspension of treatment until symptoms or radiographic signs of ARIA have resolved or permanent discontinuation of treatment. ARIA largely resolves without concomitant treatment, and there are no systematic data on potential treatments for ARIA. Given the availability of an anti-Aβ therapy, ARIA monitoring will now be implemented in routine clinical practice. The simple magnetic resonance imaging sequences used in clinical trials are likely sufficient for effective detection of cases. Increased awareness and education of ARIA among clinicians and radiologists is vital.
Key words: Alzheimer’s disease, amyloid-related imaging abnormalities, anti-amyloid beta therapy, ARIA, cerebral vasogenic edema / microhemorrhages.
Introduction
Abnormal aggregation of toxic amyloid beta (Aβ) species in the brain is believed to cause downstream pathologies that lead to neuronal death in Alzheimer’s disease (AD) (1–3); therefore, Aβ has been a primary target of therapy development for AD (4, 5). Imaging findings known as amyloid-related imaging abnormalities (ARIA) can be observed via magnetic resonance imaging (MRI) in two radiographically distinct presentations, ARIA-E and ARIA-H (Figure 1), following the treatment of AD with monoclonal antibodies (mAbs) that target Aβ (6–15).
ARIA-E refers to vasogenic edema in the brain parenchyma or sulcal effusions (Figure 1) (14, 15). ARIA-H refers to hemosiderin deposits presenting as microhemorrhages (hemorrhages <10 mm) in the brain parenchyma and/or localized superficial siderosis (located in the subarachnoid space) (Figure 1) (14, 15). The first reports of cerebral vasogenic edema and microhemorrhages associated with anti-Aβ mAb therapy were observed following bapineuzumab treatment in a phase 2 multiple ascending dose trial (6, 7, 14). ARIA has also been reported in past clinical trials of several other anti-Aβ mAb therapies, including gantenerumab, donanemab, lecanemab, and aducanumab (8-15). Aducanumab, a human immunoglobulin gamma 1 (IgG1) mAb directed against soluble and insoluble aggregated forms of Aβ, including oligomers, protofibrils, and fibrils, was granted accelerated approval by the US FDA on June 7, 2021; aducanumab is now indicated for the treatment of AD in individuals at mild cognitive impairment or mild AD dementia stages (16).
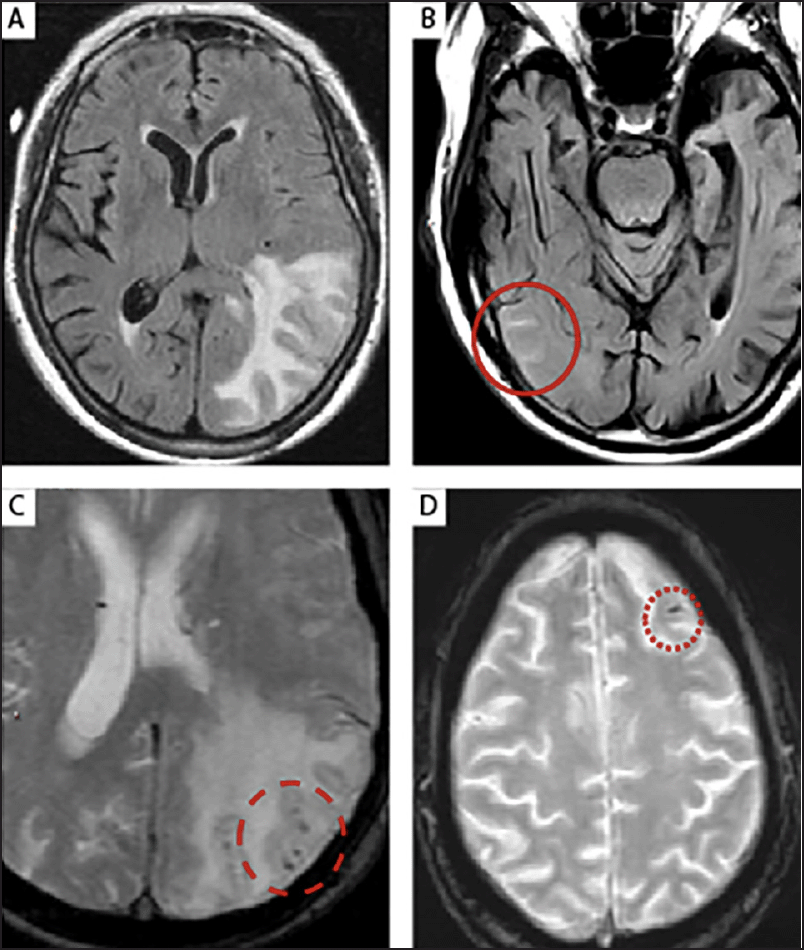
Figure 1. The MRI features of (A) parenchymal edema (ARIA-E) in the left parieto-occipital lobe (T2-FLAIR); (B) leptomeningeal effusion (ARIA-E) in several sulci within the right temporo-occipital lobe (T2-FLAIR) (solid circle); (C) microhemorrhages (ARIA-H, punctate foci of signal void on GRE/T2*) in an area of parenchymal edema (ARIA-E) (dashed circle); (D) superficial siderosis, that is a small leptomeningeal hemosiderin deposit (ARIA-H), in the left frontal lobe sulcus (dotted circle)
ARIA-E, amyloid-related imaging abnormalities due to vasogenic edema, sulcal effusions; ARIA-H, amyloid-related imaging abnormalities due to microhemorrhages, superficial siderosis; FLAIR, fluid-attenuated inversion recovery; GRE, gradient recalled echo
Although the underlying mechanisms of ARIA have not been fully elucidated in past trials, ARIA is believed to be driven by antibody-mediated breakdown of accumulated Aβ aggregates in the cerebral blood vessels and brain parenchyma (17). This breakdown of cerebrovascular Aβ may affect the structural integrity of the vessel wall, leading to increased permeability and leakage of proteinaceous fluid into surrounding tissues (17, 18). In addition, mobilization of parenchymal Aβ plaques could impair perivascular clearance and/or initiate an immune response contributing to perivascular inflammation (17, 18). Preclinical studies have shown that there may be a recovery of vessel wall integrity and an increase in effective perivascular drainage with continued treatment, and, therefore, some clinical trials have observed that the risk of ARIA is highest early in treatment and subsequently decreases in patients who continue dosing (17-20). Although ARIA-E has mainly been reported during clinical trials of Aβ-lowering agents (6, 8, 9, 11, 13, 21-25), it may also occur spontaneously in patients with AD, presumably reflecting the inflammatory component of cerebral amyloid angiopathy (26). Similarly, both microhemorrhages and superficial siderosis are also known to spontaneously occur in patients with cerebral amyloid angiopathy, independent of anti-Aβ treatment (27, 28).
Increased awareness of ARIA is needed to help ensure that suitable monitoring and management procedures accompany the current and future use of amyloid-targeting treatments in clinical practice. In this review, we summarize existing data regarding potential risk factors, detection, and management of ARIA, primarily drawn from clinical studies of bapineuzumab, gantenerumab, donanemab, lecanemab, and aducanumab.
Methods
Peer-reviewed articles were identified using PubMed and Google Scholar with the following three search strings: Search 1: (“Alzheimer Disease”[Mesh]) AND (“Amyloid related imaging abnormalities” OR “ARIA”); Search 2: “Amyloid related imaging abnormalities”; Search 3: “Alzheimer Disease”[Mesh] AND “vasogenic edema”. Relevant articles pertaining to ARIA were selected from January 2009 to November 2021; a 12-year timeframe was chosen as vasogenic edema associated with anti-Aβ mAb therapy was first observed in 2009. In addition, pertinent abstracts from recent congresses have been included to inform up-to-date ARIA management.
Results
The literature searches identified 35 primary and review papers relating to ARIA. Critical review of these papers was carried out by JB, DP, JS, and EV to identify the current gaps in knowledge. In addition, three congress abstracts were included as they provide important data pertaining to detection and management.
From these literature searches, we found that, in AD clinical studies, the incidence of ARIA-E has varied from 0.9% to 40.6% (6, 8, 9, 11, 13, 21-25, 29, 30), and the incidence of ARIA-H from 0.5% to 28.4% (11, 21-23, 25, 29, 30). The observed variability in the incidence of ARIA may be at least partly driven by differences between anti-Aβ mAbs in Aβ binding site. Clinical trials of agents that target the N-terminus of Aβ, such as aducanumab, bapineuzumab, or donanemab, have reported incidences of ARIA-E as high as 55% in apolipoprotein E (ApoE) ε4 carriers (10, 24, 29, 31). By contrast, treatment with mAbs that target the mid-domain or C-terminus of Aβ, such as solanezumab or ponezumab, has been associated with a lower incidence of ARIA (13, 32). Further, differences between anti-Aβ mAbs in affinity for different Aβ structures may contribute to variability in ARIA incidence. For example, treatment with solanezumab, which binds to soluble monomeric Aβ, was associated with a lower incidence of ARIA than were other anti-Aβ mAbs that target aggregated forms of Aβ (13).
Differences in patient populations may also play a role. ApoE ε4 non-carriers or patients with lower baseline amyloid burden have a lower risk of ARIA than ApoE ε4 carriers or those who are amyloid positive, respectively; however, earlier clinical studies may not have screened patients for ApoE ε4 status or confirmed amyloid deposition. Furthermore, differences in the frequency of MRI monitoring across clinical studies may also partially explain the variability observed. While it is important to keep trial variability in mind, this review will focus on the key findings from published clinical trials, updated MRI techniques for ARIA detection, and best practice management.
Clinical trial experience of ARIA
Risk Factors
ApoE ε4 carrier status has been shown to be a risk factor for ARIA-E and ARIA-H, with carriers having a higher incidence than non-carriers in multiple clinical trials (6, 10, 11, 25, 30, 33). ARIA-E was first reported in the phase 2 multiple ascending dose trial of bapineuzumab in 9.7% of patients (n=12/124) (6). Of those 12 patients, 10 were ApoE ε4 carriers; hence, the ARIA-E incidence was higher in carriers (13.5%; n=10/74) than non-carriers (4.3%; n=2/47) (6). Higher frequency of ARIA-E among ApoE ε4 carriers has also been observed in clinical studies of gantenerumab, donanemab, lecanemab, and aducanumab (11, 21, 23, 29, 30). Notably, the risk of ARIA-E is proportional to the number of ApoE ε4 alleles (6, 18, 25, 33). In a post-hoc analysis of three phase 2 bapineuzumab studies, there were three-fold and seven-fold increases in hazard ratios with one and two ApoE ε4 alleles, respectively, compared with non-carriers (18). In addition, ApoE ε4 homozygosity was the greatest risk for ARIA-H compared with other factors examined in a secondary analysis study of bapineuzumab (33).
The incidence of ARIA-E also seems to increase in a dose-dependent fashion with anti-Aβ mAb treatment (6, 10, 11, 21, 25, 30). Dose dependence was first noted in the phase 2 multiple ascending dose study of bapineuzumab, with incidence rates increasing from 3.2% in the 0.15 mg/kg group to 26.7% in the 2.0 mg/kg group (6). Similarly, in a central review of two phase 3 bapineuzumab studies, there was a dose-dependent increase in the incidence of ARIA-E events among non-ApoE ε4 carriers in the 0.5 mg/kg (5.6%), 1 mg/kg (13.4%), and 2 mg/kg (19.9%) groups (ApoE ε4 carriers only received the 0.5 mg/kg dose) (25). Additionally, in a phase 2 study of lecanemab, incidence of ARIA-E was higher in the 10 mg/kg monthly (9.9%) or biweekly (9.9%) groups than the 5 mg/kg monthly (2.0%) or biweekly (3.3%) groups and the 2.5 mg/kg biweekly group (1.9%) (30). Furthermore, two phase 3 studies of aducanumab also exhibited a dose-dependent increase in ARIA-E incidence between the 6 mg/kg (21.2%) and 10 mg/kg (35.2%) groups; the 3 mg/kg group, which comprised ApoE ε4 carriers only, had a higher incidence (29.5%) than the 6 mg/kg group (21).
A dose-dependent increase in ARIA-H seems to be less apparent. For example, in the bapineuzumab multiple ascending dose study, ARIA-H incidence was 24.9%, 28.0%, and 28.4% in the 0.5, 1.0, and 2.0 mg/kg non-carrier groups, respectively (25). Instead, a potential risk factor for ARIA-H may be the occurrence of ARIA-E. In an integrated safety analysis of the two phase 3 aducanumab studies, ARIA-H was stratified by ARIA-E status (21). The incidence of microhemorrhages and superficial siderosis in patients who developed ARIA-E in the 10 mg/kg group was 40.3% and 38.7%, respectively. In contrast, for patients without ARIA-E, the incidence of ARIA-H was lower and similar between the aducanumab and placebo groups (7.6% [10 mg/kg group] and 6.4% [placebo] for brain microhemorrhage, and 1.6% [10 mg/kg group] and 1.4% [placebo] for superficial siderosis) (21).
Baseline microhemorrhages were found to be another risk factor for the development of ARIA related to treatment with bapineuzumab (33, 34). A retrospective study investigated the effects of ARIA-E in two phase 3 studies of bapineuzumab; one study enrolled ApoE ε4 carriers, while the other study included non-carriers (34). The regression analysis for the non-carrier study showed that baseline microhemorrhages had no significant effect on ARIA-E occurrence; however, in the carrier study, there was an increased probability of ARIA-E in patients with baseline ARIA-H (odds ratio 2.10 [1.34, 3.29]; P=0.001) (34). Similarly, in the phase 3 aducanumab studies, the incidence of ARIA-E in the 10 mg/kg group was increased by a factor of 1.7 in patients with pre-treatment microhemorrhages compared with patients without pre-treatment microhemorrhages (21).
Considering other risk factors, given the potential pathophysiology of ARIA with regards to vessel integrity and removal of amyloid, potential links between ARIA and both cardiovascular disease and baseline amyloid load have also been studied. To date, no statistical relationship has been reported for cardiovascular factors, such as hypertension (34). Amyloid burden at baseline represents another potential risk factor for ARIA. However, in the open-label extension trials of gantenerumab, there was no relationship observed between baseline amyloid load, measured via positron emission tomography (PET) scans, and ARIA incidence (35); other trials have not explored this relationship.
The concomitant use of antithrombotic medication with anti-Aβ mAb therapy has been associated with increased risk of ARIA-H (33). Retrospective analysis of two phase 2 clinical studies and an open-label extension study of treatment with bapineuzumab found that patients who used antithrombotic agents were at greater risk of developing ARIA-H than those who did not use such medication (odds ratio 2.20 [0.99, 4.89]) (33). However, the association of antithrombotic use with risk of ARIA-H was found to be less robust than the risk posed by ApoE ε4 and baseline microhemorrhages (33). Additionally, no evidence for an association between the use of antithrombotic medication and increased hazard of ARIA-H or ARIA-E was found in the 10 mg/kg group of the phase 3 aducanumab studies (21).
The need for biomarkers to predict ARIA risk is particularly pertinent in clinical trials and in clinical practice (36). Cerebrospinal fluid (CSF) anti-Aβ autoantibodies could be a potential candidate, given that they have been proposed as a biomarker for cerebral amyloid angiopathy-related inflammation (CAA-ri) (37), which may have a similar etiology to ARIA. In biomarker sub-studies of the phase 3 bapineuzumab clinical trials, the association between ARIA-E and pathophysiological biomarkers of AD in the CSF did not predict risk for developing ARIA-E; however, a lower level of CSF Aβ42, which is indicative of cerebral amyloid accumulation, was observed in ApoE ε4 non-carriers who experienced ARIA-E than in those patients who did not develop ARIA-E (carriers and non-carriers) (38). Further research into associations between ARIA and CSF Aβ42, as well as other biomarkers, is required.
Clinical Course of ARIA
ARIA cases tend to be asymptomatic, and are usually recognized as imaging findings during routine MRI scans conducted at prespecified timepoints in clinical studies, referred to as surveillance MRI scans (10, 11, 18, 21, 22, 39). In the phase 2 multiple ascending dose study of bapineuzumab, 6 out of 12 patients remained asymptomatic after a radiographic ARIA event was identified, and the first reported symptoms of vasogenic edema included headache, confusion, vomiting, and gait disturbance (6). Similarly, in an interim analysis of the long-term extension period of a phase 1b aducanumab study, 46/185 patients experienced ARIA-E, and 61% of these patients were asymptomatic while 39% experienced symptoms similar to those reported in the bapineuzumab study (39). Regardless of the presence of symptoms, of the 46 cases of ARIA-E, 44 were transient in nature and resolved within 4–12 weeks (two cases were ongoing when the patients withdrew from the study) (39, 40). In the phase 3 studies of aducanumab, 74% of the 362 patients with ARIA-E in the highest dose group were asymptomatic (21). Similar results have been reported in randomized, placebo-controlled phase 2 studies of donanemab (78% of 36 ARIA-E cases were asymptomatic) (29) and lecanemab (89% of 46 ARIA-E cases were asymptomatic) (30), as well as phase 3 studies of gantenerumab (>80% of 55 ARIA-E cases were asymptomatic) (11) and bapineuzumab (84.5% of 103 ARIA-E cases in ApoE ε4 carriers were asymptomatic) (31). In addition, the integrated safety analysis of the aducanumab phase 3 studies found that symptoms of ARIA tended to be mild (67.7%) or moderate (28.3%) in clinical severity, while severe symptoms occurred in 4.0% of those with symptomatic ARIA in the 10 mg/kg group (21).
Most cases of ARIA occur early in the course of treatment, and the risk of ARIA appears to decrease with increased duration of exposure (11, 18, 21, 25, 31, 35). In two phase 3 bapineuzumab studies, the ARIA-E incidence in the ApoE ε4 carrier group was 5.8% between the first and second infusions, 9.0% between the second and third infusions, 4.2% between the third and fourth infusions, and 0.7% following the final infusion (25). Likewise, in the active treatment period of the phase 1b aducanumab study, first-onset ARIA-E typically occurred between the first and fifth doses of aducanumab treatment (n=31/46) (39), while in the aducanumab phase 3 studies, where treatment was initiated with a 6-month titration period, ARIA-E onset occurred largely within the first eight doses (the titration phase) of aducanumab treatment, with the probability of ARIA-E decreasing thereafter (Figure 2) (21). Moreover, in long-term extension trials of bapineuzumab, patients who received active treatment in the original studies had a lower incidence of treatment-emergent ARIA-E than did patients who switched from placebo following completion of the original trials (3.6% vs 10.7%, respectively) (22). There is some indication that the risk of ARIA-H may also decrease the longer a patient is treated with anti-Aβ mAb therapy (33); however, this is less widely reported than are ARIA-E observations and may be explained by the normal process of aging, because the frequency of cerebral microbleeds increases with age (41).
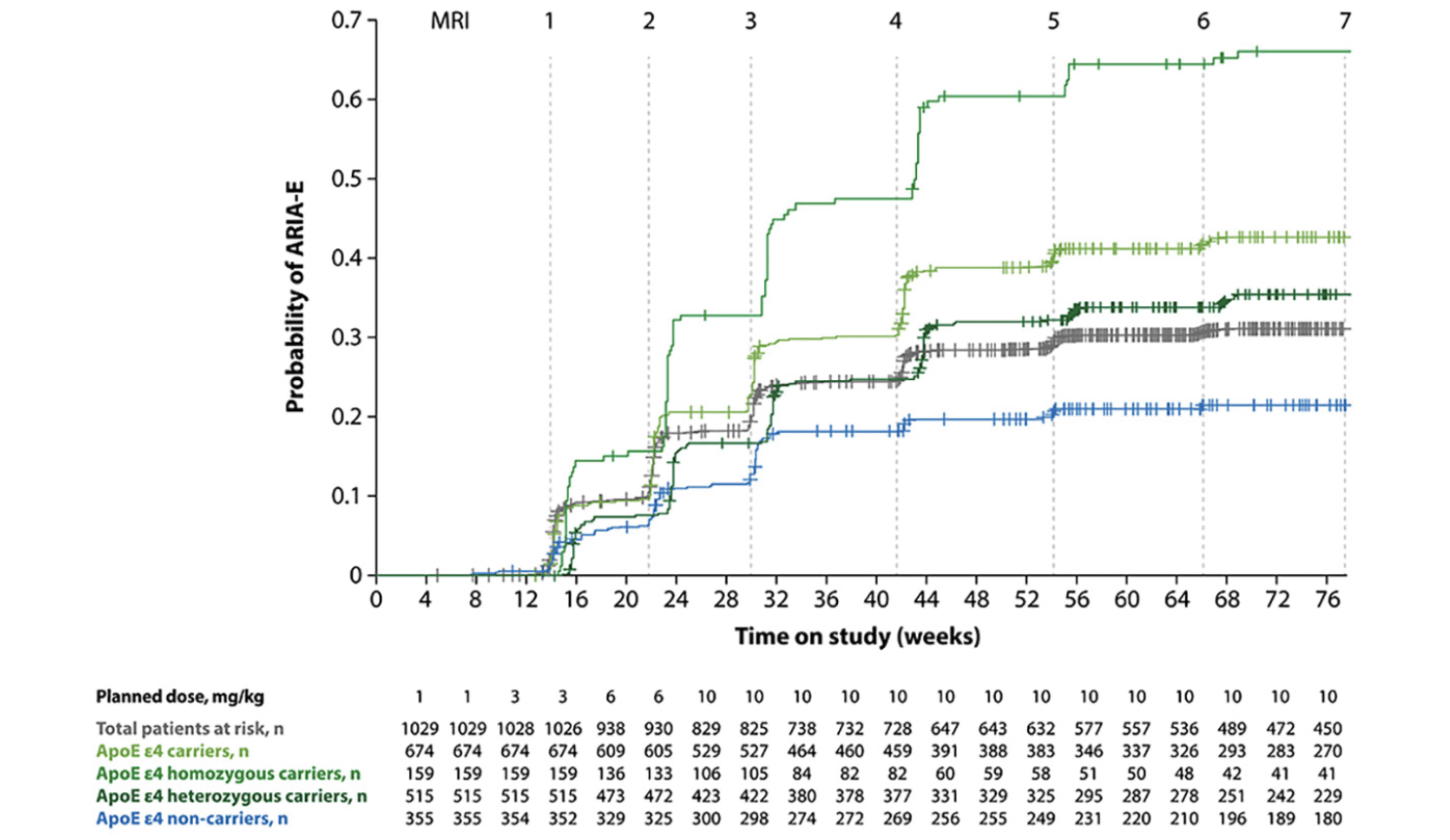
Figure 2. Kaplan-Meier plot of time to first ARIA-E event from pooled analysis of two phase 3 studies of aducanumab
Data were taken from the highest dose group (10 mg/kg) (21). Figure adapted from Salloway S, et al. Amyloid-related imaging abnormalities in 2 phase 3 studies evaluating aducanumab in early Alzheimer disease. JAMA Neurol 2022;79:13-21. https://doi.org/10.1001/jamaneurol.2021.4161 with permission from Dr. Stephen Salloway, and data verified by Biogen Inc.; ApoE, apolipoprotein E; ARIA-E, amyloid-related imaging abnormalities due to vasogenic edema, sulcal effusions
One potential hypothesis for why ARIA events are typically seen early in treatment is that there is initial loss of vessel integrity, but exposure over time leads to reconstitution of vessel wall structure as well as a resumption of more normal perivascular drainage pathways as amyloid is cleared. Evidence for this hypothesis has been gathered in a preclinical study of PDAPP mice that were immunized with weekly infusions of 3D6 (20); leakages occurred in areas with apparent Aβ plaque reduction, early in the course of treatment (detectable after 1 week of immunization), and decreased over time (20). In another similar preclinical study, a reduction in leakage with continued treatment over time was associated with improvement in vessel wall integrity (19).
Radiological Identification of ARIA
Owing to the typically transient and asymptomatic nature of ARIA, it is important to carry out routine surveillance MRI monitoring to identify and facilitate appropriate management of ARIA events. In a retrospective review of the data from three completed phase 2 bapineuzumab trials, approximately 40% (15/36) of cases of asymptomatic ARIA-E were originally missed by the local readers (18). All of the missed cases remained asymptomatic, including 13 patients who continued to receive treatment after ARIA-E occurred; asymptomatic ARIA-E recurred following additional infusions in 2 of the 13 patients who continued to receive treatment (18). This study highlighted that it was possible to dose through ARIA without substantial complications in most patients. Nevertheless, with the development of new anti-Aβ mAb treatments, awareness and appropriate recognition of ARIA by radiologists and the treating physician is critical to appropriately diagnosing ARIA following treatment initiation.
The parenchymal and sulcal forms of ARIA-E often occur together (Figure 1A and 1B) (15) and are typically diagnosed in studies as increased MRI signal hyperintensity on 2D T2 fluid-attenuated inversion recovery (FLAIR) sequences (15). High-resolution 3D T2-FLAIR sequences may provide improved conspicuity of ARIA-E-type changes while reducing potential CSF artifacts (42); however, 3D T2-FLAIR is less widely available at MRI facilities, is more sensitive to patient motion (43), and can be more time consuming than 2D T2-FLAIR (42). This could impact patient compliance with routine safety monitoring. Encouragingly, anti-Aβ therapy trials have confirmed that 2D T2-FLAIR sequences are adequate for the detection and management of ARIA-E (6, 9, 15, 18, 26, 44).
For ARIA-H, visualized on MRI as punctate foci of signal void (ie signal loss that appears as darker areas compared with the surrounding image), gradient recalled echo (GRE) / T2*-weighted sequences are currently used in trials to detect hemosiderin deposits. These deposits can result from red blood cell leakage from a vessel into adjacent parenchyma (microhemorrhage [<10 mm]) or the subarachnoid space (superficial siderosis) (Figure 1C and 1D) (15). Without GRE/T2*-weighted sequences, the small amounts of blood product cannot be detected as they are not visible on T1, T2, and FLAIR imaging owing to the use of rephasing pulse gradients (15). Considering the probable link between baseline microhemorrhage and development of ARIA (33, 34), clinical trials have excluded patients having five or more lobar hemorrhages. As such, sequences that are sensitive to hemosiderin deposits are essential in the baseline screening of patients prior to trial entry or clinical treatment. Existing literature shows that in many of the clinical trials to date, the detection of ARIA-H is based upon using GRE/T2* (9, 11, 15, 18, 26). Although susceptibility-weighted imaging (SWI) is more sensitive to hemosiderin deposits than GRE/T2* sequences (45), the use of GRE/T2* is certainly adequate. Given the increased sensitivity of SWI imaging over GRE/T2*, the impact on clinical management with anti-Aβ therapy has not been clearly defined. At the very least, it is recommended that the blood-sensitive sequence used at baseline screening, be it GRE/T2* or SWI, should be employed on all subsequent surveillance MRI scans.
When detected, ARIA-E can be graded on visual rating scales to assess severity (46-48). These severity scales assess the extent of ARIA-E and evaluate the size of edema/effusion and whether it occurs in a single region (monofocal) or in discrete separate locations (multifocal) (46-48). A simple 3-point scale, based on extent of involvement, was used in clinical studies of aducanumab and successfully categorized ARIA-E findings into mild (<5 cm and monofocal), moderate (5–10 cm and monofocal, or <10 cm and multifocal), or severe (>10 cm and mono/multifocal), with high inter-reliability between readers (21, 48). For each region of ARIA-E (contiguous region, regardless of parenchymal and/or leptomeningeal location), a measurement of the largest diameter is made (48).
In the aducanumab phase 3 studies, treatment-emergent events of ARIA-H microhemorrhage and ARIA-H superficial siderosis events were independently assessed. The severities of ARIA-H microhemorrhage and ARIA-H superficial siderosis were categorized based on the number of treatment-emergent microhemorrhages or foci of superficial siderosis: mild (≤4 new incident microhemorrhages / 1 focal area of superficial siderosis), moderate (5–9 new incident microhemorrhages / 2 focal areas of superficial siderosis), or severe (≥10 new incident microhemorrhages / >2 focal areas of superficial siderosis) (21).
In clinical practice, clinicians will likely collaborate with trained radiologists to identify the emergence of ARIA using a basic MRI protocol. An example is a scanner field strength of 1.5 or 3.0 T with the following three sequences: T2-weighted FLAIR sequence for identification of ARIA-E (14), 2D GRE/T2* for identification of ARIA-H (14), and diffusion-weighted imaging (DWI) to exclude the presence of cytotoxic edema when new areas of T2-FLAIR hyperintensity are identified (15). As ARIA-E manifests as transient vasogenic edema and is not associated with restricted diffusion / cytotoxic edema, the use of the DWI sequence is important to exclude the presence of any ischemic pathology when changes on T2-FLAIR are noted. Real-world evidence may be required to assess the number and frequency of MRI scans needed in clinical practice; planned post-authorization real-world studies of aducanumab (49) may provide opportunities for the development of standardized MRI protocols for the monitoring and management of ARIA. The optimal scan frequency may vary, based both on a given patient’s individual risk of developing ARIA and the specific anti-Aβ agent they are treated with; hence, scan frequency should be based on label recommendations and at the judgment of the prescribing clinician.
The utility of non-MRI modalities in the identification of ARIA represents a current gap in the literature. This is a key area of interest owing to the elevated risk for patients with dementia of developing comorbidities that require the implantation of medical or biostimulation devices (50). Many of these devices are contraindications of MRI, including pacemakers, vagus nerve stimulators, implantable cardioverter-defibrillators, loop recorders, insulin pumps, and cochlear implants (50). Consequently, the use of non-MRI methods for ARIA needs to be validated to ensure that adequate detection and monitoring procedures can be performed in all patients. A possible candidate is computed tomography (CT); however, the use of this modality is limited by its relatively low spatial definition and resolution in comparison with MRI (50). Therefore, CT would not be expected to detect milder forms of ARIA-E and is insensitive to the detection of microhemorrhages and siderosis. Nevertheless, if a treatment paradigm dictates treating through asymptomatic mild or moderate ARIA-E, CT may be suitable for surveillance monitoring. However, such monitoring would be deficient in detecting ARIA-H changes (microhemorrhages and siderosis) and would not assist in addressing treatment guidelines and adjustments based on development of ARIA-H changes.
Management of ARIA
The management of ARIA in clinical trials has evolved over time. Initially it was stipulated that, on detection of ARIA, anti-Aβ mAb therapy must be permanently discontinued or withheld until resolution (6, 8, 24, 31). In the phase 2 multiple ascending dose study of bapineuzumab, treatment was discontinued in 6 of 12 patients at the first detection of cerebral vasogenic edema; however, in the other six patients re-dosing was started at the lowest dose and titrated up to 50% of the originally assigned dose with no recurrence (6). Similarly, a retrospective review of the early phase 2 bapineuzumab studies found that most cases of symptomatic ARIA-E events were managed by permanent discontinuation of treatment (18). Early studies of aducanumab and gantenerumab also withdrew treatment on detection of ARIA-E (9, 44), while subsequent studies opted for reassignment to a lower dose upon resolution (11, 23). For example, in a phase 3 gantenerumab study, treatment was withheld when ARIA-E was identified; once resolved or clearly decreased and stabilized, treatment was continued at half the assigned dose (11).
In more recent clinical trials, patients with asymptomatic ARIA meeting certain radiographic and clinical criteria could continue to receive treatment but were monitored closely (23, 25, 51). In a central review of two phase 3 bapineuzumab trials, the mean ARIA-E severity scores were similar for patients who continued to receive the drug during events and those who discontinued treatment (25). However, in the non-ApoE ε4 carrier group, the severity was slightly higher for those who dosed through in the low-dose group (Supplementary Figure 1) (25). Among both carriers and non-carriers, median duration of ARIA-E was longer in patients who continued treatment than in those who discontinued treatment (25). In the phase 3 studies of aducanumab, dosing was continued following the occurrence of radiographically mild and asymptomatic cases of ARIA-E (21). Of these cases, 57.8% (93/161) remained radiographically mild and asymptomatic; radiographic severity progressed to moderate and severe in 39.8% and 0.6% of cases, respectively, and symptoms developed in 6.8% of cases (21). It has also been observed that re-dosing patients following resolution of ARIA results in recurrence in a minority of patients (8, 11, 25). For example, in the active treatment period of the phase 1b aducanumab study, only 8/46 (17.4%) patients experienced recurrent ARIA-E (with or without ARIA-H) (39). Additionally, in the phase 3 studies of aducanumab, recurrent ARIA-E was reported in 66/756 (8.7%) patients in the 3 mg/kg group, 14/392 (3.6%) patients in the 6 mg/kg group, and 109/1029 (10.6%) patients in the 10 mg/kg group (21).
Dose titration is another management step that may help mitigate the development of ARIA-E. Indeed, titration has been found to reduce the incidence of ARIA-E in ApoE ε4 carriers (10). In the phase 1b study of aducanumab, ApoE ε4 carriers who underwent a titration regimen (where the dose was increased incrementally from the lowest dose of 1 mg/kg to the highest dose of 10 mg/kg) had a lower incidence of ARIA-E (35%) than did the fixed-dose 6 mg/kg (43%) and 10 mg/kg (55%) groups (Supplementary Figure 2) (10). These key learnings were applied to the phase 3 studies of aducanumab, which employed titration, surveillance MRI monitoring, and temporary dose suspension for patients with symptoms or radiographically moderate or severe ARIA-E as risk minimization measures for ARIA (21). As such, dose titration is required for aducanumab initiation in US clinical practice (16). Further, based on the profile of ARIA events observed in the aducanumab studies, both radiographic severity and symptomatic status may inform how best to manage a patient with ARIA (Figures 3–5). Additionally, routine monitoring to identify asymptomatic ARIA events is important, and patients who are found to have ARIA should undergo careful clinical assessment (21, 23, 25). Although ARIA events may occur at any time, such events were most frequent early in treatment, and prescribers should consider ad-hoc MRI scans to assess for ARIA in patients who develop new-onset symptoms potentially consistent with ARIA.
(A) No imaging findings were observed at screening; (B) Patient developed mild asymptomatic ARIA-E after eight doses of aducanumab in a phase 1b study. At Week 30, an area of sulcal effusion (T2-FLAIR hyperintensity) with subtle potential gyral swelling, consistent with ARIA-E, was noted in the right posterior temporal-occipital lobe (circle). This was classified as mild ARIA-E as the measured area of involvement was <5 cm in diameter and monofocal (ie there were no additional foci of involvement noted elsewhere in the brain); (C) The patient continued to be dosed during the ARIA-E event, and the imaging findings resolved on a follow-up scan carried out 4 weeks later. The patient continued with dosing through Week 350 (end of study) without any recurrent ARIA events. AD, Alzheimer’s disease; ApoE, apolipoprotein E; ARIA-E, amyloid-related imaging abnormalities due to vasogenic edema, sulcal effusions; FLAIR, fluid-attenuated inversion recovery
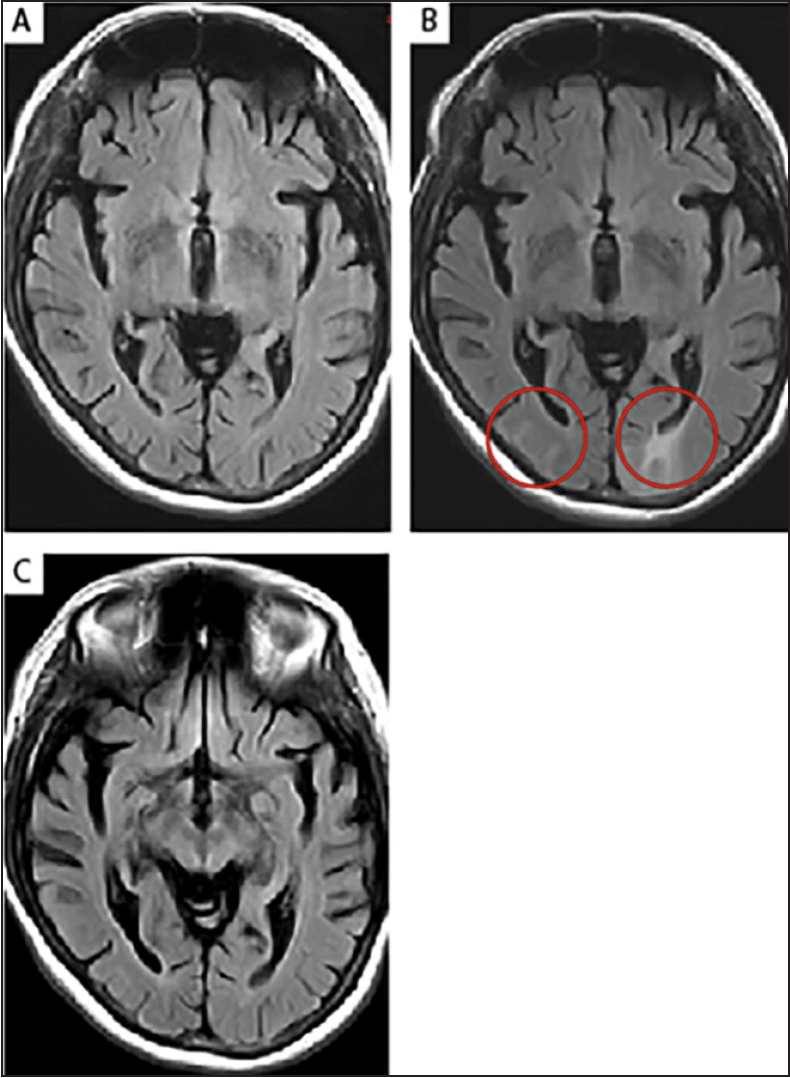
Figure 4. T2-FLAIR images of moderate ARIA-E in a 64-year-old, female ApoE ε4 carrier with prodromal AD
(A) No imaging findings were observed at screening; (B) The patient developed moderate asymptomatic ARIA-E identified at Week 6 during a brain MRI scan in a phase 1b aducanumab study. The T2-FLAIR hyperintensities (circles) were noted to involve both occipital poles. As the findings consisted of >1 area of non-contiguous involvement (multifocal), measuring <5 cm in diameter, the ARIA-E was classified as moderate. There was no associated restricted diffusion (DWI scan not shown), which is characteristic of vasogenic edema; (C) The ARIA-E imaging findings resolved 2 months later, and the patient continued with a reduced drug dose during the event, without any further recurrence of ARIA. AD, Alzheimer’s disease; ApoE, apolipoprotein E; ARIA-E, amyloid-related imaging abnormalities due to vasogenic edema, sulcal effusions; DWI, diffusion-weighted imaging; FLAIR, fluid-attenuated inversion recovery; MRI magnetic resonance imaging
A, B, and D are T2-FLAIR images. C and E are GRE/T2* images. (A) No imaging findings were observed at screening; (B) The patient developed severe asymptomatic ARIA-E identified at Week 62 during a brain MRI scan in a phase 1b aducanumab study. Extensive cerebral edema was noted involving much of the left cerebral hemisphere centered upon the left parieto-occipital lobe (T2-FLAIR hyperintensity). As the findings consisted of one area of contiguous involvement measuring >10 cm in diameter, the ARIA-E was classified as severe. With the extensive focus of parenchymal abnormality, there was note of faint associated restricted diffusion (DWI scan not shown). Associated restricted diffusion is rarely associated with ARIA-E, and when it does occur, the restricted diffusion is mild and reversible, not in keeping with the changes associated with an acute infarct; (C) At Week 62, there was also co-occurrence of several microhemorrhages (ARIA-H) using GRE/T2* sequences (circle). Despite being asymptomatic, protocol-specified actions required the drug to be withdrawn; (D) The ARIA-E imaging findings largely resolved on follow-up MRI 3 months later; (E) The microhemorrhages (circle) remain unchanged owing to the permanent tissue staining caused by hemosiderin deposition. AD, Alzheimer’s disease; ApoE, apolipoprotein E; ARIA-E, amyloid-related imaging abnormalities due to vasogenic edema, sulcal effusions; ARIA-H, amyloid-related imaging abnormalities due to microhemorrhages, superficial siderosis; DWI, diffusion-weighted imaging; FLAIR, fluid-attenuated inversion recovery; GRE, gradient recalled echo; MRI magnetic resonance imaging
As described previously, ARIA-H management in clinical trials has been determined by the cumulative number of treatment-emergent ARIA-H events. For example, in the phase 1b aducanumab study, patients continued treatment if they had mild asymptomatic ARIA-H (≤4 microhemorrhages); however, treatment was permanently discontinued for patients who experienced ≤4 microhemorrhages accompanied by moderate or severe clinical symptoms, or >4 microhemorrhages (23). This approach was also applied to the phase 3 aducanumab studies, in which moderate ARIA-H (between 5 and ≤9 microhemorrhages) was managed by dose suspension and severe ARIA-H (≥10 events) resulted in permanent discontinuation (21). Similarly, in a phase 3 gantenerumab study, if a patient experienced >4 brain microhemorrhages, treatment was discontinued, whereas treatment was continued at half the dose if a patient experienced between two and four microhemorrhages (11). Alteration of a treatment plan based on slight changes in ARIA-H counts must, however, be weighed against the fact that ARIA-H events are expected to increase with age (41).
Experience with ARIA management in clinical trials has translated into clinical recommendations. In the aducanumab US FDA prescribing information, enhanced clinical vigilance for ARIA is recommended during the first eight doses of treatment with aducanumab, particularly during titration; further, the label states that MRI should be conducted prior to the seventh and twelfth doses of aducanumab (16). Patients with radiographic ARIA should promptly undergo careful clinical evaluation, while patients who develop new-onset symptoms potentially consistent with ARIA should undergo MRI testing if indicated (16). Prescribers should then assess both the clinical and radiographic status of the patient when considering whether to continue or suspend treatment. Treatment may continue with caution following the identification of ARIA-E or mild/moderate ARIA-H; for radiographically severe ARIA-H, treatment may continue with caution after radiographic stabilization (no increase in size or number of microhemorrhages or superficial siderosis events) has been demonstrated through clinical evaluation and a follow-up MRI (16). If treatment is suspended, it is recommended that the pre-suspension dosage and titration schedule are followed upon resuming treatment (16).
Optimal treatment of ARIA events has yet to be defined. While posterior reversible encephalopathy syndrome (PRES) and CAA-ri have similar radiographic manifestations to ARIA, the treatment strategies employed for these conditions may not be relevant for the clinical management of ARIA. Owing to the large number of factors that can cause PRES, management strategies aim to address symptoms; however, in the context of drug-induced PRES, discontinuation of the causative medication usually leads to resolution of PRES (52). Unlike the majority of PRES and ARIA cases, CAA-ri is not drug induced; therefore, treatment of symptomatic cases usually involves the administration of corticosteroids and cyclophosphamide to reduce the autoantibody response to vascular Aβ and restore vessel integrity (37, 53-55). Importantly, most ARIA events in the phase 3 studies of aducanumab resolved without concomitant treatment, including those that were symptomatic or radiographically severe (21). The efficacy of steroid treatment in anti-Aβ mAb-related ARIA has not been formally studied, with only anecdotal cases treated with steroids in clinical trials at the investigator’s discretion (56). Consequently, this question deserves rigorous future study, particularly for radiographically extensive and symptomatic ARIA events.
Discussion
Well-established risk factors for ARIA include ApoE ε4 carrier status (6, 10, 11, 25, 30, 33) and high doses of anti-Aβ mAb treatment (6, 10, 11, 21, 25, 30). Clinical trials of treatment with anti-Aβ mAbs have found that ARIA is often transient and tends to occur early during treatment, with risk of ARIA decreasing over time (11, 18, 21, 25, 31, 35, 39, 40). The incidence of ARIA-H in clinical trials is generally higher among patients with ARIA-E than among those without ARIA-E (21). Furthermore, in patients without ARIA-E, the incidence of ARIA-H has been shown to be similar between treatment and placebo groups, indicating that low-level vascular events, such as ARIA-H (lobar microhemorrhages and siderosis), may occur as part of the natural course of AD (21). Further research and increased understanding of prognostic factors and biomarkers for ARIA can help to inform decisions regarding treatment strategy and monitoring procedures on an individual patient basis.
Dose titration in treatment with anti-Aβ mAbs may have mitigated the risk of ARIA-E in clinical trials, and this technique is now being recommended for real-world clinical practice in the USA (16). Moreover, cases of ARIA in clinical trials have been found to be mostly asymptomatic and have been detected through monitoring protocols with surveillance MRI scans (10, 11, 18, 21, 22, 39). As access to MRI facilities and experts who are knowledgeable in the recognition and management of ARIA in real-world practice is more limited than in clinical trials, MRI scans may be performed less frequently in real-world practice, and, as such, the detection of ARIA in the absence of symptoms may decrease compared with in clinical trials. An MRI protocol in clinical practice will need to balance detecting ARIA efficiently, as demonstrated in clinical trials, with the current capacity/readiness of MRI facilities in order to avoid overburdening the healthcare system; this is also important for the patient (reducing time spent at facilities) and payers (in terms of cost). A universal MRI protocol for use in clinical practice may streamline the detection/management of ARIA and should be validated through real-world evidence. Further research to elucidate the utility of non-MRI modalities for the detection and monitoring of ARIA may help to optimize clinical practice and further reduce the burden on MRI facilities.
With the emergence of new treatments for AD that target Aβ, training for radiologists and treating physicians is vital to increase the awareness of ARIA. As evidence from clinical trials has accumulated, it seems reasonable to infer that ARIA is both monitorable and manageable. However, the optimal detection, monitoring, and management strategies of ARIA in clinical practice may be better understood by studying the real-world use of current and future amyloid-targeting treatments.
Funding: The development of this manuscript was funded by Biogen Inc. Biogen Inc. funded medical writing support. Editorial support was provided by Robert Hodge, MSc, and Bethany Broughton, MSc, Helios Medical Communications, Cheshire, UK, which was funded by Biogen Inc. There are no other costs to declare.
Conflicts of interest: EV is an advisory consultant for several pharmaceutical companies in the field of neurodegeneration including Biogen Inc. JB, DP and JS are employees of Bioclinica Inc. (an imaging contract research organization) and advisory consultants for Biogen Inc. SC and PB are employees of Moderna Inc. CCV, CMG, and IR are employees of Biogen Inc. SC and PB were employees of Biogen Inc. during the development of this manuscript.
Authors’ contributions: JB, DP, JS, and EV conducted a critical review of the literature. All authors participated in the drafting and reviewing of the manuscript, and all authors read and approved the final manuscript.
Open Access: This article is distributed under the terms of the Creative Commons Attribution 4.0 International License (http://creativecommons.org/licenses/by/4.0/), which permits use, duplication, adaptation, distribution and reproduction in any medium or format, as long as you give appropriate credit to the original author(s) and the source, provide a link to the Creative Commons license and indicate if changes were made.
References
1. Jack CR, Bennett DA, Blennow K, Carrillo MC, Dunn B, Haeberlein SB, et al. NIA-AA Research Framework: toward a biological definition of Alzheimer’s disease. Alzheimers Dement 2018;14:535-562. https://doi.org/10.1016/j.jalz.2018.02.018.
2. Hardy JA, Higgins GA. Alzheimer’s disease: the amyloid cascade hypothesis. Science 1992;256:184-185. https://doi.org/10.1126/science.1566067.
3. Selkoe DJ, Hardy J. The amyloid hypothesis of Alzheimer’s disease at 25 years. EMBO Mol Med 2016;8:595-608. https://doi.org/10.15252/emmm.201606210.
4. Penninkilampi R, Brothers HM, Eslick GD. Safety and efficacy of anti-amyloid-β immunotherapy in Alzheimer’s disease: a systematic review and meta-analysis. J Neuroimmune Pharmacol 2017;12:194-203. https://doi.org/10.1007/s11481-016-9722-5.
5. Cummings J, Lee G, Zhong K, Fonseca J, Taghva K. Alzheimer’s disease drug development pipeline: 2021. Alzheimers Dement (N Y) 2021;7:e12179. https://doi.org/10.1002/trc2.12179.
6. Salloway S, Sperling R, Gilman S, Fox NC, Blennow K, Raskind M, et al. A phase 2 multiple ascending dose trial of bapineuzumab in mild to moderate Alzheimer disease. Neurology 2009;73:2061-2070. https://doi.org/10.1212/WNL.0b013e3181c67808.
7. Black RS, Sperling RA, Safirstein B, Motter RN, Pallay A, Nichols A, et al. A single ascending dose study of bapineuzumab in patients with Alzheimer disease. Alzheimer Dis Assoc Disord 2010;24:198-203. https://doi.org/10.1097/WAD.0b013e3181c53b00.
8. Carlson C, Siemers E, Hake A, Case M, Hayduk R, Suhy J, et al. Amyloid-related imaging abnormalities from trials of solanezumab for Alzheimer’s disease. Alzheimers Dement (Amst) 2016;2:75-85. https://doi.org/10.1016/j.dadm.2016.02.004.
9. Ferrero J, Williams L, Stella H, Leitermann K, Mikulskis A, O’Gorman J, et al. First-in-human, double-blind, placebo-controlled, single-dose escalation study of aducanumab (BIIB037) in mild-to-moderate Alzheimer’s disease. Alzheimers Dement (NY) 2016;2:169-176. https://doi.org/10.1016/j.trci.2016.06.002.
10. Budd Haeberlein S, O’Gorman J, Chiao P, Bussière T, von Rosenstiel P, Tian Y, et al. Clinical development of aducanumab, an anti-Aβ human monoclonal antibody being investigated for the treatment of early Alzheimer’s disease. J Prev Alzheimers Dis 2017;4:255-263. https://doi.org/10.14283/jpad.2017.39.
11. Ostrowitzki S, Lasser RA, Dorflinger E, Scheltens P, Barkhof F, Nikolcheva T, et al. A phase III randomized trial of gantenerumab in prodromal Alzheimer’s disease. Alzheimers Res Ther 2017;9:95. https://doi.org/10.1186/s13195-017-0318-y.
12. Cummings JL, Cohen S, van Dyck CH, Brody M, Curtis C, Cho W, et al. ABBY: A phase 2 randomized trial of crenezumab in mild to moderate Alzheimer disease. Neurology 2018;90:e1889-e1897. https://doi.org/10.1212/WNL.0000000000005550.
13. Doody RS, Thomas RG, Farlow M, Iwatsubo T, Vellas B, Joffe S, et al. Phase 3 trials of solanezumab for mild-to-moderate Alzheimer’s disease. N Engl J Med 2014;370:311-321. https://doi.org/10.1056/NEJMoa1312889.
14. Sperling R, Jack CR, Black SE, Frosch MP, Greenberg SM, Hyman BT, et al. Amyloid-related imaging abnormalities in amyloid-modifying therapeutic trials: recommendations from the Alzheimer’s Association Research Roundtable Workgroup. Alzheimers Dement 2011;7:367-385. https://doi.org/10.1016/j.jalz.2011.05.2351.
15. Barakos J, Sperling R, Salloway S, Jack C, Gass A, Fiebach JB, et al. MR imaging features of amyloid-related imaging abnormalities. AJNR Am J Neuroradiol 2013;34:1958-1965. https://doi.org/10.3174/ajnr.A3500.
16. U.S. Food & Drug Administration (FDA). Aduhelm: highlights of prescribing information (PI) 2021. https://www.biogencdn.com/us/aduhelm-pi.pdf (accessed August 19, 2021).
17. Greenberg SM, Bacskai BJ, Hernandez-Guillamon M, Pruzin J, Sperling R, van Veluw SJ. Cerebral amyloid angiopathy and Alzheimer disease – one peptide, two pathways. Nat Rev Neurol 2020;16:30-42. https://doi.org/10.1038/s41582-019-0281-2.
18. Sperling R, Salloway S, Brooks DJ, Tampieri D, Barakos J, Fox NC, et al. Amyloid-related imaging abnormalities in patients with Alzheimer’s disease treated with bapineuzumab: a retrospective analysis. Lancet Neurol 2012;11:241-249. https://doi.org/10.1016/S1474-4422(12)70015-7.
19. Zago W, Schroeter S, Guido T, Khan K, Seubert P, Yednock T, et al. Vascular alterations in PDAPP mice after anti-Aβ immunotherapy: implications for amyloid-related imaging abnormalities. Alzheimers Dement 2013;9(Suppl. 5):S105-S115. https://doi.org/10.1016/j.jalz.2012.11.010.
20. Blockx I, Einstein S, Guns P-J, Van Audekerke J, Guglielmetti C, Zago W, et al. Monitoring blood-brain barrier integrity following amyloid-β immunotherapy using gadolinium-enhanced MRI in a PDAPP mouse model. J Alzheimers Dis 2016;54:723-735. https://doi.org/10.3233/JAD-160023.
21. Salloway S, Chalkias S, Barkhof F, Burkett P, Barakos J, Purcell D, et al. Amyloid-related imaging abnormalities in 2 phase 3 studies evaluating aducanumab in early Alzheimer disease. JAMA Neurol 2022;79:13-21. https://doi.org/10.1001/jamaneurol.2021.4161.
22. Ivanoiu A, Pariente J, Booth K, Lobello K, Luscan G, Hua L, et al. Long-term safety and tolerability of bapineuzumab in patients with Alzheimer’s disease in two phase 3 extension studies. Alzheimers Res Ther 2016;8:24. https://doi.org/10.1186/s13195-016-0193-y.
23. Sevigny J, Chiao P, Bussière T, Weinreb PH, Williams L, Maier M, et al. The antibody aducanumab reduces Aβ plaques in Alzheimer’s disease. Nature 2016;537:50-56. https://doi.org/10.1038/nature19323.
24. Vandenberghe R, Rinne JO, Boada M, Katayama S, Scheltens P, Vellas B, et al. Bapineuzumab for mild to moderate Alzheimer’s disease in two global, randomized, phase 3 trials. Alzheimers Res Ther 2016;8:18. https://doi.org/10.1186/s13195-016-0189-7.
25. Ketter N, Brashear HR, Bogert J, Di J, Miaux Y, Gass A, et al. Central review of amyloid-related imaging abnormalities in two phase III clinical trials of bapineuzumab in mild-to-moderate Alzheimer’s disease patients. J Alzheimers Dis 2017;57:557-573. https://doi.org/10.3233/JAD-160216.
26. Carlson C, Estergard W, Oh J, Suhy J, Jack CR, Siemers E, et al. Prevalence of asymptomatic vasogenic edema in pretreatment Alzheimer’s disease study cohorts from phase 3 trials of semagacestat and solanezumab. Alzheimers Dement 2011;7:396-401. https://doi.org/10.1016/j.jalz.2011.05.2353.
27. Goos JD, Henneman WJ, Sluimer JD, Vrenken H, Sluimer IC, Barkhof F, et al. Incidence of cerebral microbleeds: a longitudinal study in a memory clinic population. Neurology 2010;74:1954-1960. https://doi.org/10.1212/WNL.0b013e3181e396ea.
28. Zhou C, Liu K, Yan S, Jin Y. Association between cortical superficial siderosis and dementia in patients with cognitive impairment: a meta-analysis. Front Neurol 2019;10:8. https://doi.org/10.3389/fneur.2019.00008.
29. Mintun MA, Lo AC, Duggan Evans C, Wessels AM, Ardayfio PA, Andersen SW, et al. Donanemab in early Alzheimer’s disease. N Engl J Med 2021;384:1691-1704. https://doi.org/10.1056/NEJMoa2100708.
30. Swanson CJ, Zhang Y, Dhadda S, Wang J, Kaplow J, Lai RYK, et al. A randomized, double-blind, phase 2b proof-of-concept clinical trial in early Alzheimer’s disease with lecanemab, an anti-Aβ protofibril antibody. Alzheimers Res Ther 2021;13:80. https://doi.org/10.1186/s13195-021-00813-8.
31. Salloway S, Sperling R, Fox NC, Blennow K, Klunk W, Raskind M, et al. Two phase 3 trials of bapineuzumab in mild-to-moderate Alzheimer’s disease. N Engl J Med 2014;370:322-333. https://doi.org/10.1056/NEJMoa1304839.
32. Landen JW, Andreasen N, Cronenberger CL, Schwartz PF, Börjesson-Hanson A, Östlund H, et al. Ponezumab in mild-to-moderate Alzheimer’s disease: randomized phase II PET-PIB study. Alzheimers Dement (N Y) 2017;3:393-401. https://doi.org/10.1016/j.trci.2017.05.003.
33. Arrighi HM, Barakos J, Barkhof F, Tampieri D, Jack C, Melançon D, et al. Amyloid-related imaging abnormalities-haemosiderin (ARIA-H) in patients with Alzheimer’s disease treated with bapineuzumab: a historical, prospective secondary analysis. J Neurol Neurosurg Psychiatry 2016;87:106-112. https://doi.org/10.1136/jnnp-2014-309493.
34. Brashear HR, Ketter N, Bogert J, Di J, Salloway SP, Sperling R. Clinical evaluation of amyloid-related imaging abnormalities in bapineuzumab phase III studies. J Alzheimers Dis 2018;66:1409-1424. https://doi.org/10.3233/JAD-180675.
35. Klein G, Delmar P, Voyle N, Rehal S, Hofmann C, Abi-Saab D, et al. Gantenerumab reduces amyloid-β plaques in patients with prodromal to moderate Alzheimer’s disease: a PET substudy interim analysis. Alzheimers Res Ther 2019;11:101. https://doi.org/10.1186/s13195-019-0559-z.
36. DiFrancesco JC, Longoni M, Piazza F. Anti-Aβ autoantibodies in amyloid related imaging abnormalities (ARIA): candidate biomarker for immunotherapy in Alzheimer’s disease and cerebral amyloid angiopathy. Front Neurol 2015;6:207. https://doi.org/10.3389/fneur.2015.00207.
37. Piazza F, Greenberg SM, Savoiardo M, Gardinetti M, Chiapparini L, Raicher I, et al. Anti-amyloid β autoantibodies in cerebral amyloid angiopathy-related inflammation: implications for amyloid-modifying therapies. Ann Neurol 2013;73:449-458. https://doi.org/10.1002/ana.23857.
38. Liu E, Wang D, Sperling R, Salloway S, Fox NC, Blennow K, et al. Biomarker pattern of ARIA-E participants in phase 3 randomized clinical trials with bapineuzumab. Neurology 2018;90:e877-e886. https://doi.org/10.1212/WNL.0000000000005060.
39. von Rosenstiel P, Chen T, O’Gorman J, Yee M, Castrillo-Viguera C, Chalkias S, et al. Cumulative aducanumab safety data from PRIME: a randomized, double-blind, placebo-controlled, phase 1b study. Poster presented at the 11th Clinical Trials on Alzheimer’s Disease (CTAD) Annual Meeting, Barcelona, Spain, October 24–27, 2018.
40. von Rosenstiel P, Budd Haeberlein S, Castrillo-Viguera C, Chen T, O’Gorman J, Rajagovindan R, et al. Aducanumab 48-month analyses from PRIME, a phase 1b study in patients with early Alzheimer’s disease. J Prev Alz Dis 2018;5(Suppl. 1):S168 (Abstract LBP19).
41. Poels MM, Ikram MA, van der Lugt A, Hofman A, Krestin GP, Breteler MM, et al. Incidence of cerebral microbleeds in the general population: the Rotterdam Scan Study. Stroke 2011;42:656-661. https://doi.org/10.1161/STROKEAHA.110.607184.
42. Chagla GH, Busse RF, Sydnor R, Rowley HA, Turski PA. Three-dimensional fluid attenuated inversion recovery imaging with isotropic resolution and nonselective adiabatic inversion provides improved three-dimensional visualization and cerebrospinal fluid suppression compared to two-dimensional FLAIR at 3 tesla. Invest Radiol 2008;43:547-551. https://doi.org/10.1097/RLI.0b013e3181814d28.
43. Vos SB, Micallef C, Barkhof F, Hill A, Winston GP, Ourselin S, et al. Evaluation of prospective motion correction of high-resolution 3D-T2-FLAIR acquisitions in epilepsy patients. J Neuroradiol 2018;45:368-373. https://doi.org/10.1016/j.neurad.2018.02.007.
44. Ostrowitzki S, Deptula D, Thurfjell L, Barkhof F, Bohrmann B, Brooks DJ, et al. Mechanism of amyloid removal in patients with Alzheimer disease treated with gantenerumab. Arch Neurol 2012;69:198-207. https://doi.org/10.1001/archneurol.2011.1538.
45. Docampo J, Gonzalez N, Bravo F, Sarroca D, Morales C, Bruno C. Susceptibility-weighted angiography of intracranial blood products and calcifications compared to gradient echo sequence. Neuroradiol J 2013;26:493-500. https://doi.org/10.1177/197140091302600501.
46. Bechten A, Wattjes MP, Purcell DD, Aliaga ES, Daams M, Brashear HR, et al. Validation of an MRI rating scale for amyloid-related imaging abnormalities. J Neuroimaging 2017;27:318-325. https://doi.org/10.1111/jon.12422.
47. Barkhof F, Daams M, Scheltens P, Brashear HR, Arrighi HM, Bechten A, et al. An MRI rating scale for amyloid-related imaging abnormalities with edema or effusion. AJNR Am J Neuroradiol 2013;34:1550-1555. https://doi.org/10.3174/ajnr.A3475.
48. Bracoud L, Fiebach J, Purcell D, Gaensler E, Gass A, Lindan C, et al. Validation of a simple severity scale for assessing ARIA-E. Poster presented at the Alzheimer’s Association International Conference (AAIC), London, UK, July 16–20, 2017.
49. Galvin JE, Cummings JL, Levitchi Benea M, de Moor C, Atri A, Porter VR, et al. ICARE AD-US: design of a prospective, single-arm, multicenter, noninterventional real-world study of aducanumab in the United States. Oral presentation at the Alzheimer’s Association International Conference (AAIC), Denver, CO, USA, July 25–30, 2021.
50. Pasi M, Poggesi A, Pantoni L. The use of CT in dementia. Int Psychogeriatr 2011;23(Suppl. 2):S6-S12. https://doi.org/10.1017/S1041610211000950.
51. ClinicalTrials.gov. A study of aducanumab in participants with mild cognitive impairment due to Alzheimer’s disease or with mild Alzheimer’s disease dementia to evaluate the safety of continued dosing in participants with asymptomatic amyloid-related imaging abnormalities (EVOLVE) 2020. https://clinicaltrials.gov/ct2/show/NCT03639987 (accessed September 6, 2021).
52. Fischer M, Schmutzhard E. Posterior reversible encephalopathy syndrome. J Neurol 2017;264:1608-1616. https://doi.org/10.1007/s00415-016-8377-8.
53. Kinnecom C, Lev MH, Wendell L, Smith EE, Rosand J, Frosch MP, et al. Course of cerebral amyloid angiopathy-related inflammation. Neurology 2007;68:1411-1416. https://doi.org/10.1212/01.wnl.0000260066.98681.2e.
54. Kimura A, Sakurai T, Yoshikura N, Hayashi Y, Takemura M, Takahashi H, et al. Corticosteroid therapy in a patient with cerebral amyloid angiopathy-related inflammation. J Neuroinflammation 2013;10:39. https://doi.org/10.1186/1742-2094-10-39.
55. Banerjee G, Alvares D, Bowen J, Adams ME, Werring DJ. Minimally symptomatic cerebral amyloid angiopathy-related inflammation: three descriptive case reports. J Neurol Neurosurg Psychiatry 2019;90:113-115. https://doi.org/10.1136/jnnp-2017-317347.
56. VandeVrede L, Gibbs DM, Koestler M, La Joie R, Ljubenkov PA, Provost K, et al. Symptomatic amyloid-related imaging abnormalities in an APOE ε4/ε4 patient treated with aducanumab. Alzheimers Dement (Amst) 2020;12:e12101. https://doi.org/10.1002/dad2.12101.