H.T. Zheng1, Z. Wu1, M.M. Mielke2, A.M. Murray3,4, J. Ryan1
1. School of Public Health and Preventive Medicine, Monash University, Melbourne, VIC, Australia; 2. Department of Epidemiology and Prevention, Wake Forest University School of Medicine, Winston-Salem, NC, USA; 3. Division of Geriatric and Palliative Medicine, Department of Medicine, Hennepin Healthcare, Minneapolis, Minnesota, USA; 4. Berman Center for Outcomes and Clinical Research, Hennepin Healthcare Research Institute, Minneapolis, Minnesota, USA
Corresponding Author: Joanne Ryan, School of Public Health and Preventive Medicine, Monash University, Melbourne, VIC, Australia, joanne.ryan@monash.edu
J Prev Alz Dis 2024;5(11):1189-1197
Published online July 14, 2024, http://dx.doi.org/10.14283/jpad.2024.142
Abstract
Ultrasensitive assays have been developed which enable biomarkers of Alzheimer’s disease pathology and neurodegeneration to be measured in blood. These biomarkers can aid in diagnosis, and have been used to predict risk of cognitive decline and Alzheimer’s disease. The ease and cost-effectiveness of blood collections means that these biomarkers could be applied more broadly in population-based screening, however it is critical to first understand what other factors could affect blood biomarker levels. The aim of this review was to determine the extent that sociodemographic, lifestyle and health factors have been associated with blood biomarkers of Alzheimer’s disease and neuropathology. Of the 32 studies included in this review, all but one measured biomarker levels in plasma, and age and sex were the most commonly investigated factors. The most consistent significant findings were a positive association between age and neurofilament light chain (NfL) and glial fibrillary acidic protein (GFAP), and females had higher GFAP than men. Apolipoprotein ε4 allele carriers had lower Aβ42 and Aβ42/40 ratio. Body mass index was negatively associated with GFAP and NfL, and chronic kidney disease with higher levels of all biomarkers. Too few studies have investigated other chronic health conditions and this requires further investigation. Given the potential for plasma biomarkers to enhance Alzheimer’s disease diagnosis in primary care, it is important to understand how to interpret the biomarkers in light of factors that physiologically impact blood biomarker levels. This information will be critical for the establishment of reference ranges and thus the correct interpretation of these biomarkers in clinical screening.
Key words: Alzheimer’s disease, neurodegeneration, amyloid, tau, plasma biomarkers.
Introduction
The pathway to receiving a diagnosis of Alzheimer’s disease (AD) can be long and complex, involving detailed medical assessments, laboratory tests, cognitive assessments, and neurological examinations (1, 2). A diagnosis of AD currently relies on positron emission tomography (PET) imaging or measurement of amyloid beta (Aβ) and phosphorylated Tau (p-tau) in cerebrospinal fluid (CSF) (3, 4). However, the high cost, invasiveness and relatively low availability of these specialized tools has prevented their widespread use (4). Furthermore, many individuals only receive a dementia diagnosis at an advanced stage of the disease, despite the fact that deposition of amyloid plaques and neurofibrillary tangles commences years prior to the onset of clinical symptoms (2). Early detection of AD pathology would enable timely treatments, including recruitment into clinical trials, tracking progression of the disease, and even early interventions for individuals at high risk of AD before the onset of symptoms.
Ultrasensitive assays have now been developed which permit Aβ and p-tau to be measured in blood. The findings from multiple studies have demonstrated the utility of these biomarkers to aid in the diagnosis of AD (5, 6) and to predict cognitive decline (7, 8) and the risk of AD. The ratio of Aβ42/40 appears to be a surrogate biomarker of cortical Aβ deposition, independent of clinical AD diagnosis (9, 10). Plasma p-tau 181, and p-tau 217 show high concordance with levels of brain amyloid (based on PET imaging) (9, 11), and have excellent diagnostic performance for differentiating individuals with AD from other forms of dementia (12, 13). Neurofilament light chain (NfL), a non-specific marker of neuronal injury (14, 15) has been associated with the risk of AD, vascular dementia, and other types of dementia (e.g. Frontal temporal lobe dementia (8, 16, 17). Glial fibrillary acidic protein (GFAP) is a marker of astroglial activation and neuroinflammation. Plasma GFAP levels correlate with amyloid accumulation and are higher among individuals with versus without preclinical AD pathology (18, 19). Combining these blood-based biomarker measures can also provide additional insight (20). These biomarkers may thus provide an accurate, more cost-effective and timely way to diagnose AD at the population level than CSF or PET scans, and contribute to monitoring of disease progression.
Given that these blood AD biomarkers are now available for clinical use, it is critical to better understand what factors could influence levels of these biomarkers. Some factors like the apolipoprotein E allele (APOE-ε4), the strongest genetic risk factor for AD, may affect blood biomarkers levels due to its direct impact on amyloid pathology (21). On the other hand, hepatic and renal function could modify blood biomarker concentrations through impaired peripheral clearance (22) independent of a direct link with AD (23). Indeed, a range of factors could potentially affect blood biomarker levels, as a reflection of their status as risk factors for AD, via their influence on other physiological processes, or both. Understanding the impact of these factors on the blood biomarker levels is essential for correctly interpreting the blood biomarkers for the diagnosis and prognosis of AD. The aim of this review was to determine which sociodemographic, lifestyle and health factors have been associated with blood biomarkers of AD pathology.
Method
Given the broad aim to summarise the evidence for a range of factors which may be associated with blood biomarker levels, a narrative review was undertaken. Literature were retrieved from electronic databases (e.g. PubMed, Embase and Google Scholar) up until 20th March 2024, using search terms: (biomarker*) AND (blood or plasma or serum) AND (dement* or Alzheimer* or MCI or cogniti*). The literature search was limited to human studies and articles published in English. Snowball sampling was also used (i.e. identifying studies from the reference lists of relevant articles). Authors TZ, ZU and JR assessed the eligibility of studies for inclusion.
Inclusion Criteria
Studies eligible for inclusion reported blood biomarkers Aβ42, Aβ40, the ratio of Aβ42 to Aβ40 (Aβ42/40), p-tau (e.g. 181, 217), NfL and/or GFAP, and investigated whether these biomarker levels varied according to participant socio-demographic characteristics, lifestyle factors and chronic health conditions. More specifically, we included studies that measured plasma or serum using an immunoassay or Mass Spectroscopy based platform (e.g. SIMOA, Roche elecsys, Lumipulse, IP-MS). Studies measuring t-tau were also included, although it is recognised that most current studies are no longer measuring this protein. With regards to factors that may affect biomarker levels, we focused on chronic health conditions (e.g., chronic kidney disease, diabetes, dyslipidaemia), lifestyle factors (e.g. alcohol consumption, smoking, exercise) as well as sociodemographic factors (e.g., age, sex, ethnicity and race), and body mass index (BMI). We included studies of participants across all age groups and the clinical disease spectrum with diverse characteristics and health conditions.
Exclusion Criteria
We excluded studies that only measured AD biomarkers in other biofluids (e.g. CSF), or studies that only focused on other blood biomarkers. We also excluded studies that only reported on the association between these biomarkers and cognition and/or dementia, but did not specifically report on the impact of sociodemographic or chronic conditions on the blood biomarker levels. Studies that only examined whether these biomarkers could predict future risk of disease (e.g. incident heart failure) were also not eligible for inclusion. Reviews, and abstracts published from conferences, were not included.
Data Extraction
A predesigned data extraction form, created specifically for this review, was used. For each study the following information was retrieved: study design, name (if applicable) and country in which it was conducted; basic characteristics of the study participants, namely age, sex, frequency of APOE-ε4 allele (if reported), and whether the participants had cognitive impairment or dementia; the socio-demographic and health factors examined; the blood biomarkers examined; and the main findings. TZ extracted all of the data, and this information was checked by JR and ZW.
Data Synthesis
As this is a narrative review, a meta-analysis could not be performed, and risk of bias assessment was not undertaken. The results of the included studies were instead summarised qualitatively.
Results
Table 1 shows a summary of the 32 studies which were included in this review (18, 22, 24-48). These were predominantly cohort studies conducted in the US and Canada (18 studies) or Europe (9 studies), but some were also conducted in China (3 studies) and Australia (4 studies). The size of the studies varied from 92 (less than 100) to 4444 (over 4000), with only a quarter (8 studies) having over 1000 participants. Sixteen studies examined p-tau (p-tau 181, 217), 19 examined Aβ (Aβ40, Aβ42 and Aβ42/40 ratio), 10 GFAP and 20 NfL. Eleven studies measured t-tau, and given that this biomarker is no longer commonly used, the results here will focus on the other biomarkers. All but five studies measured plasma biomarkers using the Simoa platform, and only one study measured biomarkers in serum (31).
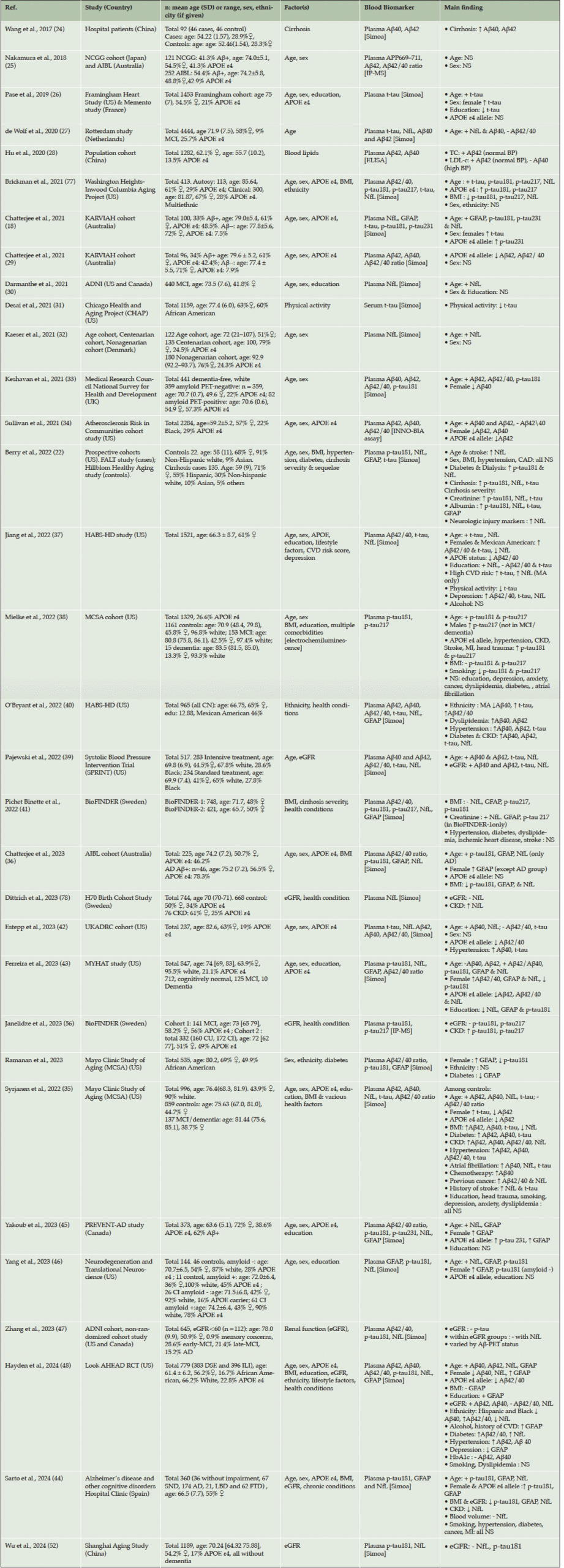
Table 1. Summary of studies reporting an association between blood AD and neurodegeneration biomarkers and sociodemographic, lifestyle or health factors
Abbreviations: Aβ: amyloid beta; AD: Alzheimer’s disease; ADNI: Alzheimer’s Disease Neuroimaging Initiative; AIBL: Australian Imaging, Biomarker & Lifestyle Flagship Study of Ageing; ALSPAC: Avon Longitudinal Study of Parents and Children; APOE-ε4: apolipoprotein E allele; APP: amyloid-β precursor protein; BMI: body mass index; BP: blood pressure; CI: cognitively impaired; CKD: chronic kidney disease; eGFR: estimated Glomerular Filtration Rate; FALT: Functional Assessment in Liver Transplantation study; FRS: Framingham Risk Score; GFAP: glial fibrillary acidic protein; HABS-HD: Health& Aging Brain study among Latino Elders, HABLE study; IP-MS: immunoprecipitation mass spectrometry; KARVIAH: The Kerr Anglican Retirement Village Initiative in Ageing Health cohort; LDL-C: serum low-density lipoprotein; MCI: mild cognitive impairment; MCSA: Mayo Clinic Study of Aging; MYHAT: Monongahela-Youghiogheny Healthy Aging Team; NAFLD: non-alcoholic fatty liver disease; NCGG: National Center for Geriatrics and Gerontology; NfL: neurofilament light chain; PET: positron emission tomography; PREVENT-AD: Pre-symptomatic Evaluation of Experimental or Novel Treatments for Alzheimer’s Disease; p-tau: phosphorylated Tau; TC: serum total cholesterol; t-tau: total Tau; UKADRC: University of Kentucky Alzheimer’s Disease Research Center.
The majority of studies assessed whether biomarker levels varied according to age and/or sex (69% and 66%, respectively). The most consistent finding was a significant positive association between NfL and age, reported in all studies that measured NfL. These studies included both cognitively unimpaired individuals, as well as individuals with cognitive impairment and dementia. Likewise, for GFAP all but two studies reported a positive correlation with age. In contrast, some studies of p-tau reported a significant positive association with age, whereas others reported no association. The findings regarding Aβ were less consistent. For example, seven studies reported no significant association between Aβ42/40 ratio and age, but four reported negative associations and two other studies reported positive associations. In terms of sex, the majority of studies reported that NfL and Aβ42/40 ratio did not vary by sex (12 and 11 studies, respectively), but multiple studies found that females had higher GFAP than males (7 studies). Four studies found that p-tau181 did not differ by sex, while three studies reported that females had higher levels and another two studies reported higher levels in men (38, 43).
A total of 14 studies examined whether the presence of the APOE-ε4 risk allele was significantly associated with plasma AD biomarker levels. The most consistent findings were a significantly lower Aβ42 (4 studies, 75%) and Aβ42/40 ratio (5 studies, 67%). In addition, there were no significant association with NfL (8 studies, 89%) and p-tau181 (6 studies, 67%). Only one study reported a lower level of NfL and 3 studies reported a higher level of p-tau181 among APOE-ε4 carriers. Only five studies assessed the level of plasma biomarkers in different racial groups. Findings suggested lower Aβ42/40 ratio and t-tau, and higher NfL in whites compared to other racial or ethnic groups (37, 40, 48), whereas other studies did not report any significant associations.
Eight studies examined the variation in levels of plasma biomarkers by BMI. Most of these studies reported a significant negative association with GFAP (67%) and NfL (75%), and to a lesser extent with p-tau (50%), but no association with Aβ42/40 ratio (67%). Apart from education level examined by 9 studies with mixed findings, other lifestyle exposures were less commonly examined, including physical activity (2 studies), smoking (4 studies) and alcohol consumption (2 studies).
In terms of chronic health conditions, the most frequently investigated has been kidney function using chronic kidney disease (CKD, 5 studies), or estimated Glomerular Filtration Rate (eGFR, 7 studies) or creatinine (2 studies), which are indicators of kidney function. The vast majority of these studies found that individuals with CKD, compared to those without, had higher biomarker levels; similarly, worse kidney function was associated with higher biomarker levels. This was found in all studies investigating NfL, Aβ42 and Aβ40, and in 80% of studies looking at p-tau181 and/or t-tau. In contrast, there was no consistent findings with regards to CKD and levels of the Aβ42/40 ratio. One study reported higher Aβ42/40 ratio in individuals with CKD (35), another no significant association (40), and eGFR was negatively associated with Aβ42/40 ratio in one study (48), but positively associated in another (39).
In terms of other chronic conditions, hypertension (8 studies) and diabetes (8 studies) have been the most frequently examined. These chronic conditions have been associated with increased Aβ42 (88%) and Aβ40 (100% of studies), but they were not associated with GFAP, p-tau181, nor Aβ42/40 ratio; and only 50% of studies reported diabetes was associated with increased NfL. The majority of other chronic conditions have only been examined by a small number of studies, and of those, few have reported significant associations.
Discussion
As a simple, accessible, and potentially scalable high-throughput test, plasma biomarkers of AD and neurodegeneration could provide the opportunity to identify individuals in the preclinical phase before dementia symptoms are apparent, potentially transforming the diagnosis of AD dementia. Blood biomarkers could thus enable interventions to begin prior to the onset of symptoms, at a stage when minimal neurodegeneration has occurred and treatments are most likely to be beneficial in slowing disease progression. However, as these biomarkers near clinical use, it is essential to understand what factors could affect the levels of these blood biomarkers, and thus need to be considered before reference ranges for these biomarkers are developed.
This review summarises existing evidence for an association between sociodemographic and lifestyle factors, and chronic conditions, on plasma biomarkers of AD and neurodegeneration. Overall, this evidence suggests that older age is associated with higher NfL and GFAP, and females had higher GFAP levels than men, and possibly higher t-tau. Carriers of the APOE-ε4 allele were frequently found to have lower Aβ42 and Aβ42/40 ratio, but most studies of these studies reported no association with p-tau181 and NfL. Higher BMI was generally associated with lower GFAP, NfL and p-tau, and better kidney function with lower NfL, p-tau181, t-tau, Aβ42 and Aβ40. Together these findings highlight the importance of considering factors such as age, sex, and health conditions when interpreting blood biomarker levels, in the context of AD diagnosis and predicting future risk, but further research is needed.
Research indicates that CKD is a strong risk factor for cognitive disorders, particularly dementia (49, 50). Similarly, patients with liver disorders appear to have a higher risk of developing AD (23, 51). The kidney and liver play an essential role in the clearance of circulating proteins from the blood. Poor renal and hepatic function can thus impact the peripheral clearance of these AD and neurodegeneration biomarkers (23, 52), resulting in increased levels in the blood. This is supported by the findings of studies that demonstrate elevated levels of these biomarkers in individuals with CKD (35, 38). While there have not many studies which have looked at liver dysfunction, the two studies that examined the effect of hepatic function also reported higher plasma biomarker levels (22, 24). Physiological processes can also account for the association between higher BMI and lower levels of plasma biomarkers. Higher BMI is linker with a greater blood volume (53, 54), which in turn, can dilute the overall concentrations of circulating proteins. Indeed, in a study of morbidly obese individuals who had lower concentrations of circulating NfL and GFAP compared to lean individuals, found that following bariatric surgery-induced weight loss, circulating levels increased (55). Ignoring factors which influence physiological processes when interpreting blood biomarker levels, could thus led to false positives or negative dementia diagnosis. Indeed, it has been shown that the difference in plasma biomarker levels (p-tau181) between participants with and without CKD exceeded the differences between biomarker levels between participants that were amyloid PET positive versus negative (38). Levels of the Aβ42/40 ratio appeared to be more robust, and less commonly affected by these physiological processes (41, 47), and examining the ratio of p-tau to t-tau may also be more beneficial in this regard (56).
The extent to which other chronic conditions could impact circulating biomarker levels is unclear. For example, the liver plays a central role in metabolic detoxification and the expression of metabolic enzymes is impaired in cirrhosis, as well as obesity and diabetes. The impact of having both cirrhosis and obesity on biomarker levels is unclear. Furthermore, several drugs can affect the production, clearance, or metabolism of proteins in the body, and thus could also lead to alterations in biomarker levels. To our knowledge, only one study has looked extensively at different medications, and found that a number of specific medications, were significant contributors to the variance in plasma biomarker levels (57). A recent study reported that sacubitril/valsartan which is used for heart failure, was associated with increased Aβ40 and Aβ42, and a reduction in Aβ42/40 ratio (58). This finding aligns with the known pharmacology of this drug, which inhibits neprilysin that is involved in clearing Aβ (59). More research is needed to better understand the potential influence of chronic health conditions, as well as drug treatments, on plasma biomarkers. Given that chronic conditions become increasing commonly as people age, and that older adults are those most susceptible to being screened for AD, this emphasizes the importance of more work in this area.
Other factors may influence measured biomarker levels because of physiological processes, as well as potentially direct impacts on neuropathological burden. The presence of the APOE-ε4 allele increases amyloidosis (21) and is the strongest genetic risk factor for late onset AD (60). This aligns with the finding that ε4 carriers were found to have lower Aβ42/40 ratio. The increase in NfL and GFAP with age, aligns with age being the strongest risk factor for neurodegeneration and results from an accumulation of cellular and molecular damage over time. However current clinically available NfL tests do not have age-specific cut-offs. Age is also a strong risk factor for AD, but not all studies have found age to be associated with an AD “risk profile” (i.e. characterised by higher tau and lower Aβ42 and Aβ42/40 ratio) (61). While Mielke et al., reported a significant positive association between age and both p-tau181 and p-tau217, they also found that this association was mainly driven by amyloid PET positive individuals (40). This indicates that p-tau might not increase with age among individuals without brain amyloid deposition.
Sex differences in plasma biomarkers have not been consistently reported, but there are several ways in which sex could affect the concentration or interpretation of blood biomarkers. Females appear to have higher blood-brain permeability than males (62), which could result in higher concentrations of brain-derived biomarkers in the blood for males. Sex differences in platelet activation (63) and blood protein concentrations could also affect the measurement (64) or levels of Aβ in the blood (65). Furthermore, differences in comorbidities between males and females (e.g. kidney function) (66) could influence sex differences in the clearance and measurement of these biomarkers. For example, one study found that males have higher levels of p-tau181 and p-tau217 (38), which contrasts with the findings of some other studies (44, 46). However, this difference was attenuated after excluding individuals with chronic kidney disease (38).
Dementia disproportionately impacts Blacks (67), who are 2-3 times more likely to develop dementia than whites (68, 69). There may be ethnic and racial differences in AD plasma biomarkers, however to date, there has been a substantial lack of diversity in these studies. A systematic review of AD fluid biomarkers levels between Blacks and whites found only one study which had measured these biomarkers in plasma (70). Of the studies included in this review, only a couple looked at possible differences based on race or ethnicity (37, 40, 48). Compared to non-Hispanic whites, higher Aβ42/40 ratio was found in Mexican Americans, Hispanics and Blacks, but lower NfL in Mexican Americans and Blacks (37, 40, 48). It should also be noted that the prevalence of many health conditions may differ by sex and ethnicity (71), and these factors may also contribute to observed sex and ethnic differences in biomarker levels.
There are limitations to this review which need to be considered. Firstly, it was a scoping review of the literature rather than a systematic review, and thus was not intended to include every study that has been published on this topic. However, a systematic search was used to capture relevant studies, and the extracted data was verified by two co-authors. The studies included in this review examined a broad range of factors, and in varying populations across different countries. It is possible however, that some factors have been examined and were not included in this review. Further, it is well recognised that individuals who volunteer to participate in research studies are not fully representative of the broader population. They are more likely to be highly educated, female and white (72). This limits the extent to which these findings can be generalised to other populations. The relatively small size of many studies included in this review mean they were under-powered to detect moderate to small effect sizes, and this could explain some of the inconsistent findings (73). Most conditions, have been inadequately studied to date, and differences in study populations, their age and cognitive status (e.g. normal, mild cognitive impairment (MCI), dementia) is also likely to influence the findings. For example, Syrjanen et al. found that only amongst controls (but not participants with MCI or dementia), women had higher t-tau levels and a lower Aβ42/40 ratio than men (35). A preliminary study involving 177 individuals showed that diet was associated with plasma biomarker levels (74). Increased intake of both ‘healthy’ and poor food choices (e.g. grains and beans, as well as fast food and red meat) was associated with increased NfL, and higher olive oil consumption was associated with lower p-tau181 (74). However, the Aβ42/40 ratio was not associated with specific foods (74). Larger studies which include a wide range of factors are needed. Finally, it is important that researchers consider the potential for confounding when measuring plasma biomarkers, and account for this appropriately. This includes firstly recognizing and capturing information on potential confounders and then adjusting for these through multivariate methods or stratification.
Conclusion
Blood biomarkers are relatively feasible and inexpensive to measure, and may enhance AD diagnosis in the primary care setting. However, it is critical to first understand how factors can affect the interpretation of these biomarkers, through an effect on physiological processes (e.g. peripheral clearance, blood volume), or amyloid pathology and neuropathological burden directly (e.g. APOE ε4), being established risk factors for AD. Some factors may influence biomarker levels through a combination of these processes. For example, chronic kidney disease could be a risk factor for dementia, but also affect renal clearance of biomarkers from the blood (75, 76). Understanding the aetiology and impact of sociodemographic, lifestyle and health factors on blood biomarkers will be essential for the establishment of reference ranges and thus the correct interpretation of these biomarkers in the clinical setting.
Conflict of interest: Anne M. Murray reports receiving consulting fees from Alkahest, Inc., and grants from the National Institute on Aging (NIA). Michelle Mielke reports funding from NIH and Alzheimer’s Association, consulting fees from Biogen, Eisai, Lilly, Merck, Roche, Siemens and Healthineers, and payments from Riche and Novo Nordisk. Joanne Ryan reports grants from NIA. Tina Zheng, Zimu Wu, and Joanne Ryan report no conflicts of interest.
Author contribution: Study concept and design: Joanne Ryan; Acquisition of data: Tina Zheng, Zimu Wu and Joanne Ryan. Analysis and interpretation of data: all authors. Preparation of manuscript draft: Tina Zheng and Joanne Ryan. Revision of draft: all authors.
Funding: Joanne Ryan is supported by a National Health and Medical Research Council Research Leader Fellowship (1135727). Anne Murray, Michelle Mielke and Joanne Ryan received funding from NIA The funders had no role in the design and conduct of the study; in the collection, analysis, and interpretation of data; in the preparation of the manuscript; or in the review or approval of the manuscript. Open Access funding enabled and organized by CAUL and its Member Institutions.
Open Access: This article is distributed under the terms of the Creative Commons Attribution 4.0 International License (http://creativecommons.org/licenses/by/4.0/), which permits use, duplication, adaptation, distribution and reproduction in any medium or format, as long as you give appropriate credit to the original author(s) and the source, provide a link to the Creative Commons license and indicate if changes were made.
References
1. Aging NIo. What Is Dementia? Symptoms, Types, and Diagnosis: National Institute on Aging; 2022 [08/04/2024]. Available from: https://www.nia.nih.gov/health/alzheimers-and-dementia/what-dementia-symptoms-types-and-diagnosis#:~:text=The%20following%20procedures%20also%20may,Brain%20scans.
2. Swaddiwudhipong N, Whiteside DJ, Hezemans FH, Street D, Rowe JB, Rittman T. Pre-diagnostic cognitive and functional impairment in multiple sporadic neurodegenerative diseases. Alzheimers Dement. 2023;19(5):1752-63.
3. Dubois B, von Arnim CAF, Burnie N, Bozeat S, Cummings J. Biomarkers in Alzheimer’s disease: role in early and differential diagnosis and recognition of atypical variants. Alzheimer’s Research & Therapy. 2023;15(1):175.
4. Palmqvist S, Zetterberg H, Mattsson N, Johansson P, Minthon L, Blennow K, et al. Detailed comparison of amyloid PET and CSF biomarkers for identifying early Alzheimer disease. Neurology. 2015;85(14):1240-9.
5. Barthélemy NR, Salvadó G, Schindler SE, He Y, Janelidze S, Collij LE, et al. Highly accurate blood test for Alzheimer’s disease is similar or superior to clinical cerebrospinal fluid tests. Nature Medicine. 2024.
6. Noda K, Lim Y, Goto R, Sengoku S, Kodama K. Cost-effectiveness comparison between blood biomarkers and conventional tests in Alzheimer’s disease diagnosis. Drug Discovery Today. 2024;29(3):103911.
7. Moscoso A, Grothe MJ, Ashton NJ, Karikari TK, Lantero Rodríguez J, Snellman A, et al. Longitudinal Associations of Blood Phosphorylated Tau181 and Neurofilament Light Chain With Neurodegeneration in Alzheimer Disease. JAMA Neurol. 2021;78(4):396-406.
8. Chouraki V, Beiser A, Younkin L, Preis SR, Weinstein G, Hansson O, et al. Plasma amyloid-β and risk of Alzheimer’s disease in the Framingham Heart Study. Alzheimers Dement. 2015;11(3):249-57.e1.
9. Doecke JD, Pérez-Grijalba V, Fandos N, Fowler C, Villemagne VL, Masters CL, et al. Total Aβ(42)/Aβ(40) ratio in plasma predicts amyloid-PET status, independent of clinical AD diagnosis. Neurology. 2020;94(15):e1580-e91.
10. Fandos N, Pérez-Grijalba V, Pesini P, Olmos S, Bossa M, Villemagne VL, et al. Plasma amyloid β 42/40 ratios as biomarkers for amyloid β cerebral deposition in cognitively normal individuals. Alzheimer’s & Dementia: Diagnosis, Assessment & Disease Monitoring. 2017;8:179-87.
11. Schindler SE, Bollinger JG, Ovod V, Mawuenyega KG, Li Y, Gordon BA, et al. High-precision plasma β-amyloid 42/40 predicts current and future brain amyloidosis. Neurology. 2019;93(17):e1647-e59.
12. Thijssen EH, La Joie R, Strom A, Fonseca C, Iaccarino L, Wolf A, et al. Plasma phosphorylated tau 217 and phosphorylated tau 181 as biomarkers in Alzheimer’s disease and frontotemporal lobar degeneration: a retrospective diagnostic performance study. Lancet Neurol. 2021;20(9):739-52.
13. Norgren N, Rosengren L, Stigbrand T. Elevated neurofilament levels in neurological diseases. Brain Res. 2003;987(1):25-31.
14. Preische O, Schultz SA, Apel A, Kuhle J, Kaeser SA, Barro C, et al. Serum neurofilament dynamics predicts neurodegeneration and clinical progression in presymptomatic Alzheimer’s disease. Nat Med. 2019;25(2):277-83.
15. Li D, Zhang L, Nelson NW, Mielke MM, Yu F. Plasma Neurofilament Light and Future Declines in Cognition and Function in Alzheimer’s Disease in the FIT-AD Trial. J Alzheimers Dis Rep. 2021;5(1):601-11.
16. Gravesteijn G, Rutten JW, Verberk IMW, Böhringer S, Liem MK, van der Grond J, et al. Serum Neurofilament light correlates with CADASIL disease severity and survival. Ann Clin Transl Neurol. 2019;6(1):46-56.
17. Benedet AL, Milà-Alomà M, Vrillon A, Ashton NJ, Pascoal TA, Lussier F, et al. Differences Between Plasma and Cerebrospinal Fluid Glial Fibrillary Acidic Protein Levels Across the Alzheimer Disease Continuum. JAMA Neurol. 2021.
18. Chatterjee P, Pedrini S, Ashton NJ, Tegg M, Goozee K, Singh AK, et al. Diagnostic and prognostic plasma biomarkers for preclinical Alzheimer’s disease. Alzheimers Dement. 2021.
19. Zetterberg H, Bendlin BB. Biomarkers for Alzheimer’s disease-preparing for a new era of disease-modifying therapies. Mol Psychiatry. 2021;26(1):296-308.
20. Rauchmann BS, Schneider-Axmann T, Perneczky R. Associations of longitudinal plasma p-tau181 and NfL with tau-PET, Aβ-PET and cognition. J Neurol Neurosurg Psychiatry. 2021;92(12):1289-95.
21. Liu CC, Liu CC, Kanekiyo T, Xu H, Bu G. Apolipoprotein E and Alzheimer disease: risk, mechanisms and therapy. Nat Rev Neurol. 2013;9(2):106-18.
22. Berry K, Asken BM, Grab JD, Chan B, Lario Lago A, Wong R, et al. Hepatic and renal function impact concentrations of plasma biomarkers of neuropathology. Alzheimers Dement (Amst). 2022;14(1):e12321.
23. Estrada LD, Ahumada P, Cabrera D, Arab JP. Liver Dysfunction as a Novel Player in Alzheimer’s Progression: Looking Outside the Brain. Front Aging Neurosci. 2019;11:174.
24. Wang YR, Wang QH, Zhang T, Liu YH, Yao XQ, Zeng F, et al. Associations Between Hepatic Functions and Plasma Amyloid-Beta Levels-Implications for the Capacity of Liver in Peripheral Amyloid-Beta Clearance. Mol Neurobiol. 2017;54(3):2338-44.
25. Nakamura A, Kaneko N, Villemagne VL, Kato T, Doecke J, Doré V, et al. High performance plasma amyloid-β biomarkers for Alzheimer’s disease. Nature. 2018;554(7691):249-54.
26. Pase MP, Beiser AS, Himali JJ, Satizabal CL, Aparicio HJ, DeCarli C, et al. Assessment of Plasma Total Tau Level as a Predictive Biomarker for Dementia and Related Endophenotypes. JAMA Neurol. 2019;76(5):598-606.
27. de Wolf F, Ghanbari M, Licher S, McRae-McKee K, Gras L, Weverling GJ, et al. Plasma tau, neurofilament light chain and amyloid-β levels and risk of dementia; a population-based cohort study. Brain. 2020;143(4):1220-32.
28. Hu N, Gao L, Jiang Y, Wei S, Shang S, Chen C, et al. The relationship between blood lipids and plasma amyloid beta is depend on blood pressure: a population-based cross-sectional study. Lipids Health Dis. 2020;19(1):8.
29. Chatterjee P, Pedrini S, Stoops E, Goozee K, Villemagne VL, Asih PR, et al. Plasma glial fibrillary acidic protein is elevated in cognitively normal older adults at risk of Alzheimer’s disease. Transl Psychiatry. 2021;11(1):27.
30. Darmanthé N, Tabatabaei-Jafari H, Cherbuin N. Combination of Plasma Neurofilament Light Chain and Mini-Mental State Examination Score Predicts Progression from Mild Cognitive Impairment to Alzheimer’s Disease within 5 Years. J Alzheimers Dis. 2021;82(3):951-64.
31. Desai P, Evans D, Dhana K, Aggarwal NT, Wilson RS, McAninch E, et al. Longitudinal Association of Total Tau Concentrations and Physical Activity With Cognitive Decline in a Population Sample. JAMA Netw Open. 2021;4(8):e2120398.
32. Kaeser SA, Lehallier B, Thinggaard M, Häsler LM, Apel A, Bergmann C, et al. A neuronal blood marker is associated with mortality in old age. Nature Aging. 2021;1(2):218-25.
33. Keshavan A, Pannee J, Karikari TK, Rodriguez JL, Ashton NJ, Nicholas JM, et al. Population-based blood screening for preclinical Alzheimer’s disease in a British birth cohort at age 70. Brain. 2021;144(2):434-49.
34. Sullivan KJ, Blackshear C, Simino J, Tin A, Walker KA, Sharrett AR, et al. Association of Midlife Plasma Amyloid-β Levels With Cognitive Impairment in Late Life: The ARIC Neurocognitive Study. Neurology. 2021;97(11):e1123-e31.
35. Syrjanen JA, Campbell MR, Algeciras-Schimnich A, Vemuri P, Graff-Radford J, Machulda MM, et al. Associations of amyloid and neurodegeneration plasma biomarkers with comorbidities. Alzheimers Dement. 2022;18(6):1128-40.
36. Chatterjee P, Pedrini S, Doecke JD, Thota R, Villemagne VL, Doré V, et al. Plasma Aβ42/40 ratio, p-tau181, GFAP, and NfL across the Alzheimer’s disease continuum: A cross-sectional and longitudinal study in the AIBL cohort. Alzheimers Dement. 2023;19(4):1117-34.
37. Jiang X, O’Bryant SE, Johnson LA, Rissman RA, Yaffe K. Association of cardiovascular risk factors and blood biomarkers with cognition: The HABS-HD study. Alzheimers Dement (Amst). 2023;15(1):e12394.
38. Mielke MM, Dage JL, Frank RD, Algeciras-Schimnich A, Knopman DS, Lowe VJ, et al. Performance of plasma phosphorylated tau 181 and 217 in the community. Nat Med. 2022;28(7):1398-405.
39. Pajewski NM, Elahi FM, Tamura MK, Hinman JD, Nasrallah IM, Ix JH, et al. Plasma amyloid beta, neurofilament light chain, and total tau in the Systolic Blood Pressure Intervention Trial (SPRINT). Alzheimers Dement. 2022;18(8):1472-83.
40. O’Bryant SE, Petersen M, Hall J, Johnson LA, Team ftH-HS. Medical comorbidities and ethnicity impact plasma Alzheimer’s disease biomarkers: Important considerations for clinical trials and practice. Alzheimer’s & Dementia. 2023;19(1):36-43.
41. Pichet Binette A, Janelidze S, Cullen N, Dage JL, Bateman RJ, Zetterberg H, et al. Confounding factors of Alzheimer’s disease plasma biomarkers and their impact on clinical performance. Alzheimers Dement. 2023;19(4):1403-14.
42. Estepp TG, Charnigo RJ, Abner EL, Jicha GA, Sudduth TL, Fardo DW, et al. Associations of potential ADRD plasma biomarkers in cognitively normal volunteers. Alzheimers Dement. 2023;19(8):3593-601.
43. Ferreira PCL, Zhang Y, Snitz B, Chang CH, Bellaver B, Jacobsen E, et al. Plasma biomarkers identify older adults at risk of Alzheimer’s disease and related dementias in a real-world population-based cohort. Alzheimers Dement. 2023;19(10):4507-19.
44. Sarto J, Esteller-Gauxax D, Tort-Merino A, Guillén N, Pérez-Millan A, Falgàs N, et al. Impact of demographics and comorbid conditions on plasma biomarkers concentrations and their diagnostic accuracy in a memory clinic cohort. J Neurol. 2024;271(4):1973-84.
45. Yakoub Y, Ashton NJ, Strikwerda-Brown C, Montoliu-Gaya L, Karikari TK, Kac PR, et al. Longitudinal blood biomarker trajectories in preclinical Alzheimer’s disease. Alzheimers Dement. 2023;19(12):5620-31.
46. Yang Z, Sreenivasan K, Toledano Strom EN, Osse AML, Pasia LG, Cosme CG, et al. Clinical and biological relevance of glial fibrillary acidic protein in Alzheimer’s disease. Alzheimers Res Ther. 2023;15(1):190.
47. Zhang B, Zhang C, Wang Y, Chen L, Qiao Y, Wang Y, et al. Effect of renal function on the diagnostic performance of plasma biomarkers for Alzheimer’s disease. Front Aging Neurosci. 2023;15:1150510.
48. Hayden KM, Mielke MM, Evans JK, Neiberg R, Molina-Henry D, Culkin M, et al. Association between Modifiable Risk Factors and Levels of Blood-Based Biomarkers of Alzheimer’s and Related Dementias in the Look AHEAD Cohort. JAR Life. 2024;13:1-21.
49. Kamide K. CKD could be a new risk factor of dementia. Hypertension Research. 2024;47(4):1090-1.
50. Kelly DM, Rothwell PM. Disentangling the Relationship Between Chronic Kidney Disease and Cognitive Disorders. Front Neurol. 2022;13:830064.
51. Abureesh M, Alkhayyat M, Abdelhay A, Badran R, Deeb L. S3253 Alzheimer’s Disease in Patients With Liver Cirrhosis: Analysis of a Large Population Registry. Official journal of the American College of Gastroenterology | ACG. 2020;115:S1701.
52. Wu J, Xiao Z, Wang M, Wu W, Ma X, Liang X, et al. The impact of kidney function on plasma neurofilament light and phospho-tau 181 in a community-based cohort: the Shanghai Aging Study. Alzheimer’s Research & Therapy. 2024;16(1):32.
53. Manouchehrinia A, Piehl F, Hillert J, Kuhle J, Alfredsson L, Olsson T, et al. Confounding effect of blood volume and body mass index on blood neurofilament light chain levels. Annals of Clinical and Translational Neurology. 2020;7(1):139-43.
54. Huff RL, Feller DD. Relation of circulating red cell volume to body density and obesity. J Clin Invest. 1956;35(1):1-10.
55. Rebelos E, Rissanen E, Bucci M, Jääskeläinen O, Honka M-J, Nummenmaa L, et al. Circulating neurofilament is linked with morbid obesity, renal function, and brain density. Scientific Reports. 2022;12(1):7841.
56. Janelidze S, Barthélemy NR, He Y, Bateman RJ, Hansson O. Mitigating the Associations of Kidney Dysfunction With Blood Biomarkers of Alzheimer Disease by Using Phosphorylated Tau to Total Tau Ratios. JAMA Neurol. 2023;80(5):516-22.
57. Bouteloup V, Pellegrin I, Dubois B, Chene G, Planche V, Dufouil C, et al. Explaining the Variability of Alzheimer Disease Fluid Biomarker Concentrations in Memory Clinic Patients Without Dementia. Neurology. 2024;102(8):e209219.
58. Brum WS, Docherty KF, Ashton NJ, Zetterberg H, Hansson O, McMurray JJV, et al. Effect of Neprilysin Inhibition on Alzheimer Disease Plasma Biomarkers: A Secondary Analysis of a Randomized Clinical Trial. JAMA Neurology. 2024;81(2):197-200.
59. Grimm MO, Mett J, Stahlmann CP, Haupenthal VJ, Zimmer VC, Hartmann T. Neprilysin and Aβ Clearance: Impact of the APP Intracellular Domain in NEP Regulation and Implications in Alzheimer’s Disease. Front Aging Neurosci. 2013;5:98.
60. Riaz M, Huq A, Ryan J, Orchard SG, Tiller J, Lockery J, et al. Effect of APOE and a polygenic risk score on incident dementia and cognitive decline in a healthy older population. Aging Cell. 2021;20(6):e13384.
61. Palmqvist S, Stomrud E, Cullen N, Janelidze S, Manuilova E, Jethwa A, et al. An accurate fully automated panel of plasma biomarkers for Alzheimer’s disease. Alzheimer’s & Dementia. 2023;19(4):1204-15.
62. Parrado-Fernández C, Blennow K, Hansson M, Leoni V, Cedazo-Minguez A, Björkhem I. Evidence for sex difference in the CSF/plasma albumin ratio in ~20 000 patients and 335 healthy volunteers. J Cell Mol Med. 2018;22(10):5151-4.
63. Johnson M, Ramey E, Ramwell PW. Sex and age differences in human platelet aggregation. Nature. 1975;253(5490):355-7.
64. Weaving G, Batstone GF, Jones RG. Age and sex variation in serum albumin concentration: an observational study. Ann Clin Biochem. 2016;53(Pt 1):106-11.
65. Carbone MG, Pagni G, Tagliarini C, Imbimbo BP, Pomara N. Can platelet activation result in increased plasma Aβ levels and contribute to the pathogenesis of Alzheimer’s disease? Ageing Res Rev. 2021;71:101420.
66. Carrero JJ, Hecking M, Chesnaye NC, Jager KJ. Sex and gender disparities in the epidemiology and outcomes of chronic kidney disease. Nat Rev Nephrol. 2018;14(3):151-64.
67. Matthews KA, Xu W, Gaglioti AH, Holt JB, Croft JB, Mack D, et al. Racial and ethnic estimates of Alzheimer’s disease and related dementias in the United States (2015-2060) in adults aged ≥65 years. Alzheimers Dement. 2019;15(1):17-24.
68. Morris JC, Schindler SE, McCue LM, Moulder KL, Benzinger TLS, Cruchaga C, et al. Assessment of Racial Disparities in Biomarkers for Alzheimer Disease. JAMA Neurol. 2019;76(3):264-73.
69. Weuve J, Barnes LL, Mendes de Leon CF, Rajan KB, Beck T, Aggarwal NT, et al. Cognitive Aging in Black and White Americans: Cognition, Cognitive Decline, and Incidence of Alzheimer Disease Dementia. Epidemiology. 2018;29(1):151-9.
70. Chaudhry A, Rizig M. Comparing fluid biomarkers of Alzheimer’s disease between African American or Black African and white groups: A systematic review and meta-analysis. J Neurol Sci. 2021;421:117270.
71. Vlassoff C. Gender differences in determinants and consequences of health and illness. J Health Popul Nutr. 2007;25(1):47-61.
72. Scanlon JK, Wofford L, Fair A, Philippi D. Predictors of Participation in Clinical Research. Nurs Res. 2021;70(4):289-97.
73. Hajian-Tilaki K. Sample size estimation in epidemiologic studies. Caspian J Intern Med. 2011;2(4):289-98.
74. Dempsey DA, Deardorff R, Apostolova LG, Brosch JR, Clark DG, Farlow MR, et al. Mediterranean Diet Foods are Associated with Plasma Biomarkers of Alzheimer’s Disease and Related Dementias. Alzheimer’s & Dementia. 2023;19(S14):e080051.
75. Sedaghat S, Ji Y, Hughes TM, Coresh J, Grams ME, Folsom AR, et al. The Association of Kidney Function with Plasma Amyloid-β Levels and Brain Amyloid Deposition. Journal of Alzheimer’s Disease. 2023;92:229-39.
76. Stocker H, Beyer L, Trares K, Perna L, Rujescu D, Holleczek B, et al. Association of Kidney Function With Development of Alzheimer Disease and Other Dementias and Dementia-Related Blood Biomarkers. JAMA Network Open. 2023;6(1):e2252387-e.
77. Brickman AM, Manly JJ, Honig LS, Sanchez D, Reyes-Dumeyer D, Lantigua RA, et al. Plasma p-tau181, p-tau217, and other blood-based Alzheimer’s disease biomarkers in a multi-ethnic, community study. Alzheimers Dement. 2021;17(8):1353-64.
78. Dittrich A, Ashton NJ, Zetterberg H, Blennow K, Zettergren A, Simrén J, et al. Association of Chronic Kidney Disease With Plasma NfL and Other Biomarkers of Neurodegeneration: The H70 Birth Cohort Study in Gothenburg. Neurology. 2023;101(3):e277-e88.
© The Authors 2024