C. Hooper1, P. De Souto Barreto1, M. Pahor2, M. Weiner3, B. Vellas1,4
1. Gérontopôle, Department of Geriatrics, CHU Toulouse, Purpan University Hospital, Toulouse, France; 2. Department of Aging and Geriatric Research, Institute on Aging, College of Medicine, University of Florida, 2004 Mowry Road, Gainesville, Florida; 3. University of California San Francisco, School of Medicine, 4150 Clement Street, San Francisco, California. USA; 4. INSERM UMR 1027, Toulouse, France.
Corresponding Author: Claudie Hooper, 1Gérontopôle, Department of Geriatrics, CHU Toulouse, Purpan University Hospital, Toulouse, France, Tel : +33 (5) 61 77 64 25,
Fax : +33 (5) 61 77 64 75 claudie28@yahoo.com
J Prev Alz Dis 2018;5(1):78-84/ONLINE EXCLUSIVE
Published online June 13, 2017, http://dx.doi.org/10.14283/jpad.2017.19
Abstract
Significant research attention has focussed on the identification of nutraceutical agents for the prevention of cognitive decline as a natural means of cognitive preservation in the elderly. There is some evidence for a reduction of brain omega 3 polyunsaturated fatty acids (n-3 PUFAs) in normal aging and in Alzheimer’s disease. n-3 PUFAs exhibit anti-inflammatory and anti-amyloidogenic properties as well as being able to reduce tau phosphorylation. Many observational studies have demonstrated a link between n-3 PUFAs and cognitive aging, and some, but not all, randomized controlled trials have demonstrated a benefit of n-3 PUFA supplementation on cognition, particularly in those subjects with mild cognitive impairment. The identification of a biomarker that reflects n-3 PUFA intake over time and consequent tissue levels is required. In this narrative review we discuss the evidence associating red blood cell membrane n-3 PUFAs with cognitive function and structural brain changes associated with Alzheimer’s disease.
Key words: Docosahexaenoic acid, omega 3 polyunsaturated fatty acids, cognitive decline, Alzheimer’s disease, red blood cell.
Introduction
Alzheimer’s disease
Alzheimer’s disease (AD) is a neurodegenerative disorder of aging characterised by progressive memory loss, cognitive impairment and the inability to carry out functional activities of daily living (1). AD is characterised pathologically by the presence of cerebral β-amyloid (Aβ), neurofibrillary tangles composed of hyper-phosphorylated tau and neurodegeneration (2). The presence of the apolipoprotein E (ApoE) ε4 allele is the main genetic risk factor associated with sporadic disease, which is the predominant form of AD (3). Other factors that have been reported to influence the onset of AD include diet as well as physical and mental activity (4, 5).
n-3 PUFAs
Omega 3 polyunsaturated fatty acids (n-3 PUFAs) are dietary factors that have received significant research attention in relation to their beneficial effects on cognitive decline. The main n-3 PUFAs used in the body are docosahexaenoic acid (DHA) and eicosapentaenoic acid (EPA) and are mostly obtained from the consumption of oily fish or through dietary supplementation (6). DHA and EPA can also be synthesised to a limited extent from α-linolenic acid (ALA) obtained from plant oils (6). Docasapentaenoic acid (DPA) is one of the less extensively studied n-3 PUFAs, which nonetheless plays a role in influencing health outcomes that are responsive to DHA and EPA (7, 8).
DHA is the major fatty acid in neuronal membranes (30 %) and is enriched in synaptosomal membranes (9). DHA is involved in multiple inter-related brain functions including cell membrane fluidity, signal transduction and neurotransmission (10–12). DHA is thought to be the main n-3 PUFA involved in cerebral metabolism and as a consequence has been most extensively studied. The major facilitator superfamily domain-containing protein 2a (Mfsd2a) has recently been identified as the major transporter for the uptake of DHA (in the lysophosphatidylcholine form) into the brain (13). EPA also crosses the blood brain barrier (BBB), although through an as yet unidentified mechanism (14) and is present in brain tissue, albeit at considerably lower concentrations (15). EPA and DHA exhibit overlapping and unique biological roles (15, 16) and EPA serves as a precursor to DHA in the biosynthetic pathway.
n-3 PUFAs and AD
Cerebral DHA levels are known to be deficient in AD specifically in brain regions associated with disease (17,18) and DHA is decreased in the brain in normal human aging (19). There are a number of possible explanations which could account for the reduction in cerebral DHA including insufficient dietary intake, reduced BBB transit, increased neuronal death or genetic variability in delta-5 desaturase (FADS1) and delta-6 desaturase (FADS2); enzymes involved in the rate limiting steps of DHA and EPA synthesis (20). Increased levels of plasma fatty acid binding proteins, which are known to increase with age (21, 22), could also account for reduced cerebral DHA and/or increased oxidative damage to fatty acids caused by free radicals associated with age and inflammation could lead to diminished cerebral levels (23–25).
Many observational studies have demonstrated a link between n-3 PUFAs and cognitive aging related to AD (26). Some randomised controlled trials (RCTs) have also demonstrated a benefit of n-3 PUFA supplementation, particularly in terms of immediate recall, attention and processing speed in patients with mild cognitive impairment (MCI), but not in those with AD or in healthy subjects (27). MCI if present in conjunction with cerebral Aβ represents the prodromal stage of AD and offers a window of opportunity for therapeutic intervention. Identifying elderly patients at risk of cognitive decline is important to enable timely treatment before AD pathology becomes irreversible; as such n-3 PUFAs might offer a potential well tolerated, inexpensive treatment in the early stages of AD.
There is increasing evidence to suggest that n-3 PUFAs play a role in the disease mechanisms associated with AD. n-3 PUFAs have been shown to promote long term potentiation (LTP), a mechanism underpinning functional plasticity the basis of learning and memory, in cell culture and animal models (11,28). There is also evidence to suggest that DHA confers neuro-protection in part through the direct inhibition of tau phosphorylation (29,30). Moreover, DHA and EPA have been shown to alter amyloid precursor protein (APP) processing in favour of reduced Aβ production (31–35).
In terms of inflammation, n-3 PUFAs are known to displace omega 6 polyunsaturated fatty acids (n-6 PUFAs) from cell membranes resulting in the production of more benign eicosanoids that possess less potent inflammatory and thrombotic effects and are less efficacious vasoconstrictors (6,36). Thus, changing DHA/EPA concentrations in cell membranes might serve to ameliorate cerebral inflammation, which is known to fuel AD pathology (37). In addition, increased membrane n-3 PUFA content might reduce the incidence of stroke, which is associated with an increased incidence of AD (38, 39) and vascular dementia (40). n-3 PUFAs also specifically suppress the expression of pro-inflammatory cytokines and promote microglial phagocytosis of Aβ and increase neurotrophin production (41, 42). n-3 PUFAs skew macrophage/microglial polarisation towards an M2 anti-inflammatory phenotype indicative of tissue repair (41, 43) and provide the building blocks for the production of E and D series resolvins, protectins and maresins – collectively known as specialized pro-resolving mediators (SPMs) (44). These mediators are involved in the resolution and termination of the inflammatory response, which was originally thought to be a passive process. Diminished levels of SPMs are found in the hippocampus (31) and in the entorhinal cortex in AD (45), which is in accordance with the chronic pro-inflammatory micro-environment associated with AD brain (46). Interestingly, SPMs have been shown to promote microglial phagocytosis of Aβ and reduce M1 microglial cell surface marker expression in addition to possessing neuroprotective properties (45). Thus, n-3 PUFA dietary supplementation might serve to reduce cerebral inflammation both directly and indirectly through the production of SPMs thereby limiting bystander damage to neurons and subsequent neurodegeneration. Furthermore, pro-inflammatory microglial activation has been purported to provide the link between Aβ plaques and hyper-phosphorylated tau (37, 47), therefore promoting microglial M2 anti-inflammatory activity and phagocytosis of Aβ through the increased consumption of n-3 PUFAs might serve to ameliorate tau pathology, the best clinical correlate of neurodegeneration (48,4 9), hence curtailing AD-related symptoms.
Rationale for this narrative review
A number of systematic reviews and meta-analyses have addressed the effects of n-3 PUFA supplementation on measures of cognitive aging associated with AD and MCI (27, 50–52). Furthermore, clinical signs of AD and MCI have frequently been correlated with plasma DHA and/or EPA levels (9, 53, 54). However, plasma levels of fatty acids (free, cholesteryl esters or phospholipid bound) reflect recent dietary intake over a time frame of a few days and therefore do not represent a true picture of steady-state n-3 PUFA levels (55). RBC fatty acid concentration might represent a more reliable measurement of dietary habits and nutritional status; considering that fatty acids are stable in RBC membranes for up to 3 months corresponding to the lifespan of a RBC (55). RBC fatty acid concentrations have also been shown to reflect tissue concentrations (56). Thus, in this narrative review we aim to present the literature whereby RBC n-3 PUFA levels have been investigated in association with cognitive function and brain structure related to AD. A systematic review was out of the scope of this study.
Methods
In April, 2016, a search without time date span limitation was performed in Pubmed. A search strategy was implemented using key words in an ‘AND’ combination to identify studies pertaining to RBC n-3 PUFA (red blood cell, erythrocyte, omega 3 or PUFA) and cognitive function and brain structure related to AD (cogniti*, atrophy, dementia, ‘mild cognitive impairment’, Alzheimer*). Titles were subjected to screening followed by an assessment of relevant abstracts by one author. Articles had to meet the following inclusion criteria: (a) quantitative assessment of RBC n-3 PUFA levels reported in the article and (b) assessment of cognitive outcomes or brain structure. An updating literature search was performed in February 2017 to add recent key references. Only manuscripts written in English were included. Note, the definition of RBC ‘total n-3 PUFAs’ differs between studies, therefore the precise fatty acids measured in a specific study and designated as ‘total n-3 PUFAs’ are specified where relevant in parentheses in this review. Of note, the studies described here should be compared with caution considering the divergent roles that different n-3 PUFAs play in biology, particularly, DHA and EPA. The specific terms describing n-3 PUFAs should not be considered as interchangeable.
Results and discussion
Study characteristics
As a result of the initial and updating literature searches 14 articles were included in this review (Table 1) (57–70). Of the studies 12 were of observational design and 2 were RCTs. Four studies were performed in the USA, two studies were performed in France, Taiwan, Australia and Scotland and one study was undertaken in Germany and another Canada. Sample sizes varied between 46 to 2157 participants with subjects ranging from cognitively normal to demented with a diagnosis of AD.
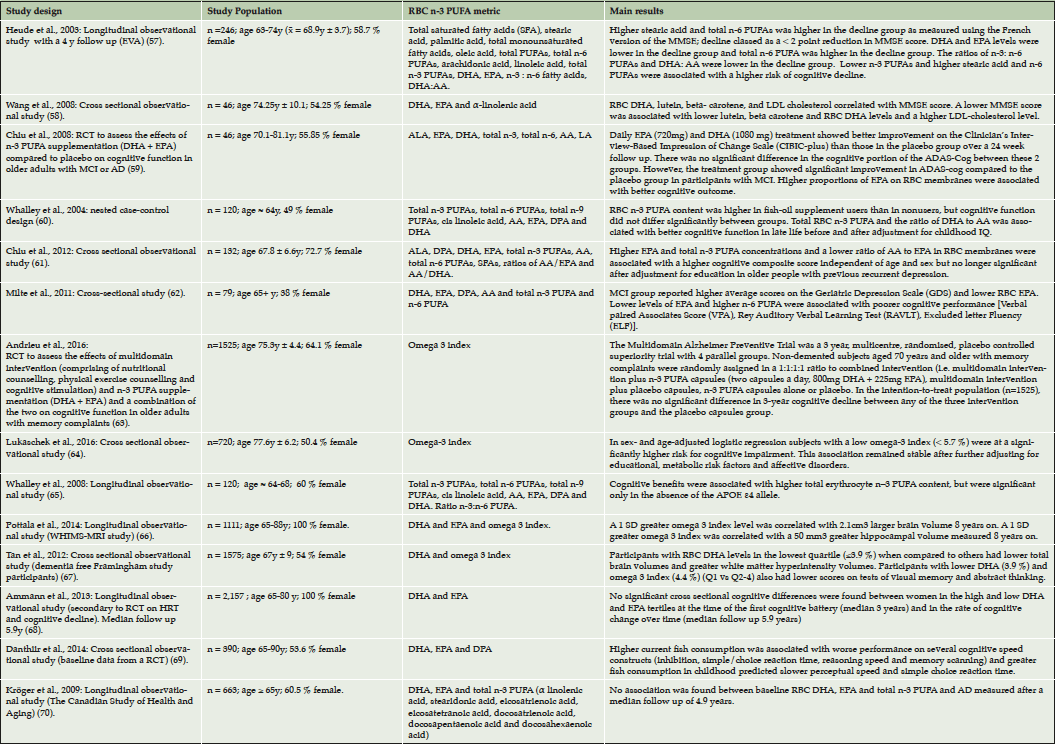
Table 1. Summary of the main findings of the studies relating RBC n-3 PUFAs to parameters associated with cognitive function and brain structure in relation to AD
References listed sequentially as mentioned in the main text.
Studies relating to RBC n-3 PUFAs and cognition
The Etude du Vieillissement Artérial (EVA) was the first study to relate fatty acid composition of RBC membranes with cognitive decline. This observational study consisted of 246 cognitively normal participants and demonstrated an inverse association between cognitive decline over a four year period and the ratio of n-3 to n-6 PUFAs in RBC membranes measured at baseline (57). It was suggested that higher levels of RBC n-6 PUFAs could reflect a deficiency in brain levels of n-3 PUFAs, which in turn could affect cognition. Subsequent studies have also associated RBC PUFA with cognition. A cross-sectional study investigating nutritional biomarkers of AD comprising 46 subjects has shown that a reduction in RBC DHA correlates with a reduction in MMSE score (58). Higher RBC EPA has been associated with better ADAS-cog scores in a small RCT comprising 46 participants with either MCI or AD (59). A longitudinal observational analysis of 350 participants born in 1936 has reported that those who take fish oil supplements have significantly greater RBC n-3 PUFA levels and that total RBC n-3 PUFA (exact fatty acids not specified) and the ratio of DHA to AA were associated with better cognitive performance in late life before and after adjustment for childhood IQ (60). Higher RBC EPA levels as well as total RBC n-3 PUFAs (defined as the sum of ALA, EPA, DHA and DPA) were positively associated with cognitive composite scores in a cross sectional study of 132 participants formerly suffering from depression at risk of cognitive decline (61). This association was independent of age and sex but, was no longer significant after adjustment for education. This may reflect a wider association between cognitive function and healthy lifestyle related to higher education. Moreover, a cross-sectional study of 79 participants has reported that patients with MCI have lower RBC EPA and higher depressive scores (62).
We have recently completed a three year RCT known as the Multidomain Alzheimer Preventive Trial (MAPT), which was designed to assess the effects of DHA (800 mg) and EPA (to a maximum of 225mg), multidomain intervention (comprising of nutritional counselling, physical exercise counselling and cognitive training) and a combination of the two on alterations in cognitive function in frail subjects with memory complaints aged over seventy (4). In the main analysis of MAPT, no significant effects of the interventions were found on cognition after adjustment for multiple testing (63). Exploratory sub-group analysis showed that participants on n-3 PUFA supplementation with a low omega-3 index (DHA + EPA ≤ 4.83 %, representing the lowest quartile of omega 3 index distribution) at baseline showed a trend towards less cognitive decline over 36 months in comparison to subjects on placebo with low baseline omega-3 index. Furthermore, exploratory within group analysis of MAPT data has shown that participants in the placebo group with a low omega-3 index at baseline underwent significant cognitive decline over 36 months, whereas those in the placebo group with a higher omega-3 index (quartiles 2-4) remained stable. Consistent with our findings, results from the KORA (KOoperativen Gesundheitsforschung in der Region Augsburg)-Age study have shown a cross-sectional association between low omega 3 index (< 5.7 %) and cognitive impairment in an elderly population of 720 subjects with cognitive status ranging from cognitively normal to suspected dementia (64).
n-3 PUFAs and the role of ApoE ε4
A longitudinal observational study comprising 120 participants has demonstrated cognitive benefits associated with higher total RBC n-3 PUFAs (exact fatty acids not specified), but only in the absence of the ApoE ε4 allele (65). In this study cognitive performance at the age of around 64 and cognitive changes between approximately 64 to 68 years of age were related to RBC n-3 PUFA on recruitment and ApoE ε4 allele status. This report was an extension of the study by Whalley et al., 2004, described above and used their original sample of patients born in 1936 (60). In accordance with these findings, some studies have shown that the protective cognitive effects of n-3 PUFAs are seen only in ApoE ε4 non-carriers (71,72) and that plasma DHA levels show little change in ApoE ε4 carriers despite supplementation (73). In contrast, a recent study has shown the opposite, demonstrating that n-3 PUFA consumption is related to slower cognitive decline in ApoE ε4 carriers (74). Thus, the effects of ApoE ε4 on n-3 PUFA status and cognition warrants further research investigation.
Studies relating to RBC n-3 PUFAs and structural brain changes
The Women’s Health Initiative Memory Study (WHIMS) Magnetic Resonance Imaging (MRI) a longitudinal observational study comprising 1111 dementia-free participants demonstrated that a higher baseline RBC omega 3 index correlated with larger total brain volumes and hippocampal volume measured 8 years on (66). Furthermore, a recent cross-sectional analysis of 1575 dementia-free participants from the Framingham Offspring cohort has shown that subjects in the lowest quartile with regard to RBC DHA levels exhibit lower total brain volumes but, greater white matter hyperintensity volumes without any significant changes in hippocampal volume (67). Participants in the lowest quartile of the Framingham Offspring cohort with regard to RBC DHA and omega 3 index also had lower scores in tests of visual memory, executive function and abstract thinking. Interestingly, the presence of increased white matter hyperintensities in the latter study is suggestive of dementia of a more vascular nature.
RBC n-3 PUFAs and cognition: the negative study findings
An observational analysis performed on 2157 cognitively-intact elderly women enrolled on an RCT designed to investigate the effects of hormone therapy on normal cognitive aging failed to show an association of baseline RBC DHA and EPA levels with cognitive change. Cognitive assessments were performed at a median of 3 years after randomization and then annually with a median follow up of 5.9 years (68). Another cross-sectional observational study of 390 cognitively normal older adults from the Older People, Omega 3 and Cognitive Health trial (EPOCH) found no evidence to support the hypothesis that higher RBC n-3 PUFA (exact fatty acids not specified) exert a beneficial effect on baseline cognitive performance (69). In fact, the results suggest a small negative effect of fish intake in childhood and in older age on older-age cognitive function that was tentatively hypothesised to be attributable to higher concentrations of the environmental neurotoxin methyl-mercury in the fish. Furthermore, the Canadian Study of Health and Ageing (CSHA), a longitudinal observational study comprising 663 participants demonstrated there to be no association between baseline total RBC n-3 PUFAs, (defined as the sum of RBC EPA, DHA, DPA, ALA, stearidonic acid, eicosatrienoic acid and eicosatetranoic acid), RBC DHA or RBC EPA and the incidence of AD measured after a median follow up period of 4.9 years (70). However, exposure to n-3 PUFAs assessed approximately 5 years before a diagnosis of dementia may not reflect a true representation of dietary habits. A stable and sustained regular intake of n-3 PUFAs is probably required to confer mental health benefits in the elderly.
Conclusion
Here we present evidence associating RBC n-3 PUFAs with cognitive function and brain structure associated with AD. The measurement of RBC n-3 PUFAs as a biomarker of n-3 PUFA status is advantageous considering its stability over time. There is also some evidence from RCTs to suggest that the administration of n-3 PUFAs (DHA and EPA) can prevent cognitive decline. Further studies aimed at clarifying the relationship between RBC n-3 PUFAs and cognitive impairment are required. Future research should aim to specifically explore the effects of n-3 PUFA supplementation on cognitive decline and brain structure in n-3 PUFA deficient elderly subjects. It might be that n-3 PUFA supplementation per se is not beneficial in the prevention of disease, but rather re-establishing homoeostatic levels in deficient elderly subjects is pivotal for the preservation of cognition. The mechanisms through which n-3 PUFAs operate, (neurodegenerative versus vascular and the involvement of inflammation) also deserves further research attention specifically as a function of ApoE ε4 status and FADS haplotype. The expression of such genetic variants could account for the differential responses to n-3 PUFA supplementation observed between studies. In fact, we are at present in the process of planning such a trial as an offspring study to MAPT in order to address some of these important as yet unanswered questions.
Funding/support: There was no external funding for this study.
Conflicts of Interest: The authors declare no conflict of interest. There were no financial relationships with any organizations that might have an interest in the submitted work or no other relationships or activities that could appear to have influenced the submitted work.
References
1. von Strauss E, Viitanen M, De Ronchi D, Winblad B, Fratiglioni L. Aging and the occurrence of dementia: findings from a population-based cohort with a large sample of nonagenarians. Arch Neurol. 1999 May;56(5):587–92.
2. Hardy J, Allsop D. Amyloid deposition as the central event in the aetiology of Alzheimer’s disease. Trends Pharmacol Sci. 1991 Oct;12(10):383–8.
3. Corder EH, Saunders AM, Strittmatter WJ, Schmechel DE, Gaskell PC, Small GW, et al. Gene dose of apolipoprotein E type 4 allele and the risk of Alzheimer’s disease in late onset families. Science. 1993 Aug 13;261(5123):921–3.
4. Vellas B, Carrie I, Gillette-Guyonnet S, Touchon J, Dantoine T, Dartigues JF, et al. MAPT Study: A Multidomain approach for preventing Alzheimer’s disease: Design and baseline data. J Prev Alzheimers Dis. 2014 Jun;1(1):13–22.
5. Köbe T, Witte AV, Schnelle A, Lesemann A, Fabian S, Tesky VA, et al. Combined omega-3 fatty acids, aerobic exercise and cognitive stimulation prevents decline in gray matter volume of the frontal, parietal and cingulate cortex in patients with mild cognitive impairment. NeuroImage. 2015 Oct 1;
6. Simopoulos AP. Omega-3 fatty acids in inflammation and autoimmune diseases. J Am Coll Nutr. 2002 Dec;21(6):495–505.
7. Rissanen T, Voutilainen S, Nyyssönen K, Lakka TA, Salonen JT. Fish oil-derived fatty acids, docosahexaenoic acid and docosapentaenoic acid, and the risk of acute coronary events: the Kuopio ischaemic heart disease risk factor study. Circulation. 2000 Nov 28;102(22):2677–9.
8. Oda E, Hatada K, Katoh K, Kodama M, Nakamura Y, Aizawa Y. A case-control pilot study on n-3 polyunsaturated fatty acid as a negative risk factor for myocardial infarction. Int Heart J. 2005 Jul;46(4):583–91.
9. Kyle DJ, Schaefer E, Patton G, Beiser A. Low serum docosahexaenoic acid is a significant risk factor for Alzheimer’s dementia. Lipids. 1999;34 Suppl:S245.
10. Guixà-González R, Javanainen M, Gómez-Soler M, Cordobilla B, Domingo JC, Sanz F, et al. Membrane omega-3 fatty acids modulate the oligomerisation kinetics of adenosine A2A and dopamine D2 receptors. Sci Rep. 2016;6:19839.
11. McGahon BM, Martin DS, Horrobin DF, Lynch MA. Age-related changes in synaptic function: analysis of the effect of dietary supplementation with omega-3 fatty acids. Neuroscience. 1999;94(1):305–14.
12. Lin Q, Ruuska SE, Shaw NS, Dong D, Noy N. Ligand selectivity of the peroxisome proliferator-activated receptor alpha. Biochemistry (Mosc). 1999 Jan 5;38(1):185–90.
13. Nguyen LN, Ma D, Shui G, Wong P, Cazenave-Gassiot A, Zhang X, et al. Mfsd2a is a transporter for the essential omega-3 fatty acid docosahexaenoic acid. Nature. 2014 May 22;509(7501):503–6.
14. Freund Levi Y, Vedin I, Cederholm T, Basun H, Faxén Irving G, Eriksdotter M, et al. Transfer of omega-3 fatty acids across the blood-brain barrier after dietary supplementation with a docosahexaenoic acid-rich omega-3 fatty acid preparation in patients with Alzheimer’s disease: the OmegAD study. J Intern Med. 2014 Apr;275(4):428–36.
15. Dyall SC. Long-chain omega-3 fatty acids and the brain: a review of the independent and shared effects of EPA, DPA and DHA. Front Aging Neurosci. 2015;7:52.
16. Song C, Shieh C-H, Wu Y-S, Kalueff A, Gaikwad S, Su K-P. The role of omega-3 polyunsaturated fatty acids eicosapentaenoic and docosahexaenoic acids in the treatment of major depression and Alzheimer’s disease: Acting separately or synergistically? Prog Lipid Res. 2016 Jan 4;62:41–54.
17. Prasad MR, Lovell MA, Yatin M, Dhillon H, Markesbery WR. Regional membrane phospholipid alterations in Alzheimer’s disease. Neurochem Res. 1998 Jan;23(1):81–8.
18. Söderberg M, Edlund C, Kristensson K, Dallner G. Fatty acid composition of brain phospholipids in aging and in Alzheimer’s disease. Lipids. 1991 Jun;26(6):421–5.
19. McNamara RK, Liu Y, Jandacek R, Rider T, Tso P. The aging human orbitofrontal cortex: decreasing polyunsaturated fatty acid composition and associated increases in lipogenic gene expression and stearoyl-CoA desaturase activity. Prostaglandins Leukot Essent Fatty Acids. 2008 May;78(4–5):293–304.
20. Ameur A, Enroth S, Johansson A, Zaboli G, Igl W, Johansson ACV, et al. Genetic adaptation of fatty-acid metabolism: a human-specific haplotype increasing the biosynthesis of long-chain omega-3 and omega-6 fatty acids. Am J Hum Genet. 2012 May 4;90(5):809–20.
21. Niizeki T, Takeishi Y, Takabatake N, Shibata Y, Konta T, Kato T, et al. Circulating levels of heart-type fatty acid-binding protein in a general Japanese population: effects of age, gender, and physiologic characteristics. Circ J Off J Jpn Circ Soc. 2007 Sep;71(9):1452–7.
22. Pelsers MM, Chapelle JP, Knapen M, Vermeer C, Muijtjens AM, Hermens WT, et al. Influence of age and sex and day-to-day and within-day biological variation on plasma concentrations of fatty acid-binding protein and myoglobin in healthy subjects. Clin Chem. 1999 Mar;45(3):441–3.
23. Nourooz-Zadeh J, Liu EH, Yhlen B, Anggård EE, Halliwell B. F4-isoprostanes as specific marker of docosahexaenoic acid peroxidation in Alzheimer’s disease. J Neurochem. 1999 Feb;72(2):734–40.
24. Montine TJ, Neely MD, Quinn JF, Beal MF, Markesbery WR, Roberts LJ, et al. Lipid peroxidation in aging brain and Alzheimer’s disease. Free Radic Biol Med. 2002 Sep 1;33(5):620–6.
25. Bazan NG. Omega-3 fatty acids, pro-inflammatory signaling and neuroprotection. Curr Opin Clin Nutr Metab Care. 2007 Mar;10(2):136–41.
26. Cederholm T, Salem N, Palmblad J. ω-3 fatty acids in the prevention of cognitive decline in humans. Adv Nutr Bethesda Md. 2013 Nov;4(6):672–6.
27. Mazereeuw G, Lanctôt KL, Chau SA, Swardfager W, Herrmann N. Effects of ω-3 fatty acids on cognitive performance: a meta-analysis. Neurobiol Aging. 2012 Jul;33(7):1482.e17-29.
28. Minogue AM, Lynch AM, Loane DJ, Herron CE, Lynch MA. Modulation of amyloid-beta-induced and age-associated changes in rat hippocampus by eicosapentaenoic acid. J Neurochem. 2007 Nov;103(3):914–26.
29. Ma Q-L, Yang F, Rosario ER, Ubeda OJ, Beech W, Gant DJ, et al. Beta-amyloid oligomers induce phosphorylation of tau and inactivation of insulin receptor substrate via c-Jun N-terminal kinase signaling: suppression by omega-3 fatty acids and curcumin. J Neurosci Off J Soc Neurosci. 2009 Jul 15;29(28):9078–89.
30. Green KN, Martinez-Coria H, Khashwji H, Hall EB, Yurko-Mauro KA, Ellis L, et al. Dietary docosahexaenoic acid and docosapentaenoic acid ameliorate amyloid-beta and tau pathology via a mechanism involving presenilin 1 levels. J Neurosci Off J Soc Neurosci. 2007 Apr 18;27(16):4385–95.
31. Lukiw WJ, Cui J-G, Marcheselli VL, Bodker M, Botkjaer A, Gotlinger K, et al. A role for docosahexaenoic acid–derived neuroprotectin D1 in neural cell survival and Alzheimer disease. J Clin Invest. 2005 Oct 1;115(10):2774–83.
32. Grimm MOW, Kuchenbecker J, Grösgen S, Burg VK, Hundsdörfer B, Rothhaar TL, et al. Docosahexaenoic acid reduces amyloid beta production via multiple pleiotropic mechanisms. J Biol Chem. 2011 Apr 22;286(16):14028–39.
33. Perez SE, Berg BM, Moore KA, He B, Counts SE, Fritz JJ, et al. DHA diet reduces AD pathology in young APPswe/PS1 Delta E9 transgenic mice: possible gender effects. J Neurosci Res. 2010 Apr;88(5):1026–40.
34. Lim GP, Calon F, Morihara T, Yang F, Teter B, Ubeda O, et al. A diet enriched with the omega-3 fatty acid docosahexaenoic acid reduces amyloid burden in an aged Alzheimer mouse model. J Neurosci Off J Soc Neurosci. 2005 Mar 23;25(12):3032–40.
35. Yang X, Sheng W, Sun GY, Lee JC-M. Effects of fatty acid unsaturation numbers on membrane fluidity and α-secretase-dependent amyloid precursor protein processing. Neurochem Int. 2011 Feb;58(3):321–9.
36. Keli SO, Feskens EJ, Kromhout D. Fish consumption and risk of stroke. The Zutphen Study. Stroke J Cereb Circ. 1994 Feb;25(2):328–32.
37. McGeer PL, McGeer EG. The amyloid cascade-inflammatory hypothesis of Alzheimer disease: implications for therapy. Acta Neuropathol (Berl). 2013 Oct;126(4):479–97.
38. Zhou J, Yu J-T, Wang H-F, Meng X-F, Tan C-C, Wang J, et al. Association between stroke and Alzheimer’s disease: systematic review and meta-analysis. J Alzheimers Dis JAD. 2015;43(2):479–89.
39. de la Torre JC. How do heart disease and stroke become risk factors for Alzheimer’s disease? Neurol Res. 2006 Sep;28(6):637–44.
40. Viswanathan A, Rocca WA, Tzourio C. Vascular risk factors and dementia: how to move forward? Neurology. 2009 Jan 27;72(4):368–74.
41. Hjorth E, Zhu M, Toro VC, Vedin I, Palmblad J, Cederholm T, et al. Omega-3 fatty acids enhance phagocytosis of Alzheimer’s disease-related amyloid-β42 by human microglia and decrease inflammatory markers. J Alzheimers Dis JAD. 2013;35(4):697–713.
42. Moon D-O, Kim K-C, Jin C-Y, Han M-H, Park C, Lee K-J, et al. Inhibitory effects of eicosapentaenoic acid on lipopolysaccharide-induced activation in BV2 microglia. Int Immunopharmacol. 2007 Feb;7(2):222–9.
43. Chang HY, Lee H-N, Kim W, Surh Y-J. Docosahexaenoic acid induces M2 macrophage polarization through peroxisome proliferator-activated receptor γ activation. Life Sci. 2015 Jan 1;120:39–47.
44. Spite M, Serhan CN. Novel lipid mediators promote resolution of acute inflammation: impact of aspirin and statins. Circ Res. 2010 Nov 12;107(10):1170–84.
45. Zhu M, Wang X, Hjorth E, Colas RA, Schroeder L, Granholm A-C, et al. Pro-Resolving Lipid Mediators Improve Neuronal Survival and Increase Aβ42 Phagocytosis. Mol Neurobiol. 2016 May;53(4):2733–49.
46. McGeer PL, McGeer EG. Inflammation of the brain in Alzheimer’s disease: implications for therapy. J Leukoc Biol. 1999 Apr;65(4):409–15.
47. Zotova E, Nicoll JA, Kalaria R, Holmes C, Boche D. Inflammation in Alzheimer’s disease: relevance to pathogenesis and therapy. Alzheimers Res Ther. 2010 Jan 22;2(1):1.
48. Gómez-Isla T, Hollister R, West H, Mui S, Growdon JH, Petersen RC, et al. Neuronal loss correlates with but exceeds neurofibrillary tangles in Alzheimer’s disease. Ann Neurol. 1997 Jan;41(1):17–24.
49. Giannakopoulos P, Herrmann FR, Bussière T, Bouras C, Kövari E, Perl DP, et al. Tangle and neuron numbers, but not amyloid load, predict cognitive status in Alzheimer’s disease. Neurology. 2003 May 13;60(9):1495–500.
50. Huang TL. Omega-3 fatty acids, cognitive decline, and Alzheimer’s disease: a critical review and evaluation of the literature. J Alzheimers Dis JAD. 2010;21(3):673–90.
51. Sydenham E, Dangour AD, Lim W-S. Omega 3 fatty acid for the prevention of cognitive decline and dementia. Cochrane Database Syst Rev. 2012 Jun 13;(6):CD005379.
52. Issa AM, Mojica WA, Morton SC, Traina S, Newberry SJ, Hilton LG, et al. The efficacy of omega-3 fatty acids on cognitive function in aging and dementia: a systematic review. Dement Geriatr Cogn Disord. 2006;21(2):88–96.
53. Conquer JA, Tierney MC, Zecevic J, Bettger WJ, Fisher RH. Fatty acid analysis of blood plasma of patients with Alzheimer’s disease, other types of dementia, and cognitive impairment. Lipids. 2000 Dec;35(12):1305–12.
54. Corrigan FM, Van Rhijn AG, Ijomah G, McIntyre F, Skinner ER, Horrobin DF, et al. Tin and fatty acids in dementia. Prostaglandins Leukot Essent Fatty Acids. 1991 Aug;43(4):229–38.
55. Arab L. Biomarkers of fat and fatty acid intake. J Nutr. 2003 Mar;133 Suppl 3:925S–932S.
56. Harris WS, Sands SA, Windsor SL, Ali HA, Stevens TL, Magalski A, et al. Omega-3 fatty acids in cardiac biopsies from heart transplantation patients: correlation with erythrocytes and response to supplementation. Circulation. 2004 Sep 21;110(12):1645–9.
57. Heude B, Ducimetière P, Berr C, EVA Study. Cognitive decline and fatty acid composition of erythrocyte membranes–The EVA Study. Am J Clin Nutr. 2003 Apr;77(4):803–8.
58. Wang W, Shinto L, Connor WE, Quinn JF. Nutritional biomarkers in Alzheimer’s disease: the association between carotenoids, n-3 fatty acids, and dementia severity. J Alzheimers Dis JAD. 2008 Feb;13(1):31–8.
59. Chiu C-C, Su K-P, Cheng T-C, Liu H-C, Chang C-J, Dewey ME, et al. The effects of omega-3 fatty acids monotherapy in Alzheimer’s disease and mild cognitive impairment: a preliminary randomized double-blind placebo-controlled study. Prog Neuropsychopharmacol Biol Psychiatry. 2008 Aug 1;32(6):1538–44.
60. Whalley LJ, Fox HC, Wahle KW, Starr JM, Deary IJ. Cognitive aging, childhood intelligence, and the use of food supplements: possible involvement of n-3 fatty acids. Am J Clin Nutr. 2004 Dec;80(6):1650–7.
61. Chiu C-C, Frangou S, Chang C-J, Chiu W-C, Liu H-C, Sun I-W, et al. Associations between n-3 PUFA concentrations and cognitive function after recovery from late-life depression. Am J Clin Nutr. 2012 Feb;95(2):420–7.
62. Milte CM, Sinn N, Street SJ, Buckley JD, Coates AM, Howe PRC. Erythrocyte polyunsaturated fatty acid status, memory, cognition and mood in older adults with mild cognitive impairment and healthy controls. Prostaglandins Leukot Essent Fatty Acids. 2011 Jun;84(5–6):153–61.
63. Andrieu S, Guyonnet S, Coley N, Cantet C, Bonnefoy M, Bordes S, et al. Effect of long-term omega 3 polyunsaturated fatty acid supplementation with or without multidomain intervention on cognitive function in elderly adults with memory complaints (MAPT): a randomised, placebo-controlled trial. Lancet Neurol. 2017 Mar 27;
64. Lukaschek K, von Schacky C, Kruse J, Ladwig K-H. Cognitive Impairment Is Associated with a Low Omega-3 Index in the Elderly: Results from the KORA-Age Study. Dement Geriatr Cogn Disord. 2016;42(3–4):236–45.
65. Whalley LJ, Deary IJ, Starr JM, Wahle KW, Rance KA, Bourne VJ, et al. n-3 Fatty acid erythrocyte membrane content, APOE varepsilon4, and cognitive variation: an observational follow-up study in late adulthood. Am J Clin Nutr. 2008 Feb;87(2):449–54.
66. Pottala JV, Yaffe K, Robinson JG, Espeland MA, Wallace R, Harris WS. Higher RBC EPA + DHA corresponds with larger total brain and hippocampal volumes: WHIMS-MRI study. Neurology. 2014 Feb 4;82(5):435–42.
67. Tan ZS, Harris WS, Beiser AS, Au R, Himali JJ, Debette S, et al. Red blood cell ω-3 fatty acid levels and markers of accelerated brain aging. Neurology. 2012 Feb 28;78(9):658–64.
68. Ammann EM, Pottala JV, Harris WS, Espeland MA, Wallace R, Denburg NL, et al. ω-3 fatty acids and domain-specific cognitive aging: secondary analyses of data from WHISCA. Neurology. 2013 Oct 22;81(17):1484–91.
69. Danthiir V, Hosking D, Burns NR, Wilson C, Nettelbeck T, Calvaresi E, et al. Cognitive performance in older adults is inversely associated with fish consumption but not erythrocyte membrane n-3 fatty acids. J Nutr. 2014 Mar;144(3):311–20.
70. Kröger E, Verreault R, Carmichael P-H, Lindsay J, Julien P, Dewailly E, et al. Omega-3 fatty acids and risk of dementia: the Canadian Study of Health and Aging. Am J Clin Nutr. 2009 Jul;90(1):184–92.
71. Huang TL, Zandi PP, Tucker KL, Fitzpatrick AL, Kuller LH, Fried LP, et al. Benefits of fatty fish on dementia risk are stronger for those without APOE epsilon4. Neurology. 2005 Nov 8;65(9):1409–14.
72. Barberger-Gateau P, Raffaitin C, Letenneur L, Berr C, Tzourio C, Dartigues JF, et al. Dietary patterns and risk of dementia: the Three-City cohort study. Neurology. 2007 Nov 13;69(20):1921–30.
73. Plourde M, Vohl M-C, Vandal M, Couture P, Lemieux S, Cunnane SC. Plasma n-3 fatty acid response to an n-3 fatty acid supplement is modulated by apoE epsilon4 but not by the common PPAR-alpha L162V polymorphism in men. Br J Nutr. 2009 Oct;102(8):1121–4.
74. van de Rest O, Wang Y, Barnes LL, Tangney C, Bennett DA, Morris MC. APOE ε4 and the associations of seafood and long-chain omega-3 fatty acids with cognitive decline. Neurology. 2016 May 4;